Abstract
This paper provides a general overview of the Bishop Tuff eruption, one of the most prominent volcanic eruptions in recent history. Introduced with a brief explanation of volcanism, the paper focuses on the characteristics of the eruption, as well as what interpretations we can make about the nature of the eruption based on the evidence found in the products of the eruption.
Introduction
760,000 years ago, North America experienced one of its largest eruptions in its history. A colossal volcano unleashed its power, covering much of the western U.S. in ash and leaving a 450km2 depression in the Earth’s surface (Bailey 1976). The caldera is located on the eastern edge of the Sierra Nevada Mountains and the western edge of Glass Mountain, with Mono Lake centered 28 miles to the north. The edge of the caldera is clearly outlined on all sides (Sierras in west, White Mt. in east, Deadman summit in north) except for the southeast boundary. While the eruption occurred relatively recently in geologic terms, this area has been under constant change for millions of years. Roughly 150 million years ago, the oceanic Farallon plate began to subduct under the continental North American plate. This subduction continued for more than 100 million years until the Farallon was completely underneath the North American plate. As it dropped below the continental plate, it carried with it volatiles such as water and gases. As the plate dove further, temperatures got higher and the volatiles leaked from the Farallon, causing the surrounding continental rocks to melt. Some of these rocks came to the surface and erupted in volcanic events and some cooled under the earth as a batholith of granite. The granite formed in pockets separate from each other, or plutons, and as a result geologists can now find many types of granite, each with slightly different chemical makeups, in the Sierran batholith (Hill 2006). The caldera also lies on the western edge of the Basin and Range region. Characterized by the repeating pattern of a valley (graben) between two mountain ranges (horsts), much of the western U.S. features this topography. This region was formed when extensional forces at normal faults running north-south caused one block of crust to rise while an adjacent block dropped. Gradually, towering horsts grew, separated by a graben. These faults are active in the Long Valley area, serving as the medium for sometimes hundreds of earthquakes a day (Hill, Bailey 1985). The faults also serve as pathways for magma is gather under the area. While it is unknown why so much magma has gathered in the Long Valley area specifically, it is accepted that Basin and Range related extension is a contributing factor.
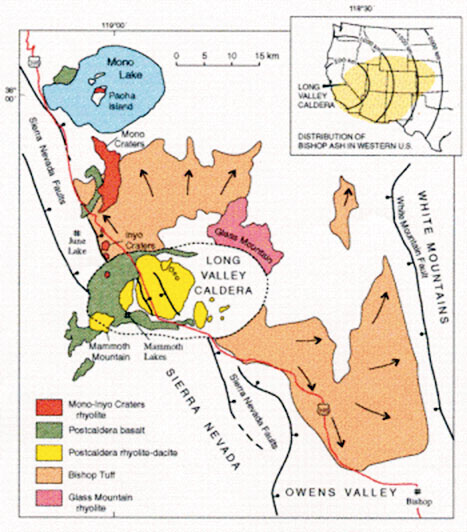
Simplified Geologic Map of Long Valley Caldera. Taken from http://volcanoes.usgs.gov/vsc/images/image_mngr/100-199/img155_350w_399h.gif
The water and volatiles present in the subducting Fallaron seafloor plate were superheated by the mantle and rose upward out of the downward-moving plate and into the crustal plate above, heating the rock to the point of melting, forming pockets of magma. These either cooled very slowly into plutons of intrusive granite or were erupted by the Sierran Arc volcanoes (Hill, 2006). These plutons of granitic rock now form the backbone of the Sierra Nevada range, and are collectively known as the Sierra Nevada Batholith.
The Fallaron plate eventually disappeared beneath the continent, exposing the underside of the crust to the mantle once more. Deformation occurred as the crust was heated, causing the thinning and stretching that created the current Basin and Range region to the east of the Sierra Nevada. Some researchers doubt that the Sierra Nevada’s uplift was influenced by this same event, however it is important to the Sierra region as it provides some geological and climactic context.
Volcanism in Long Valley
Under the earth, magma flows and is kept liquid by the enormous pressure and high temperatures. The magma contains dissolved volatiles, such as gases and H2O, which are also kept in a liquid state because of the pressure. As magma moves upwards towards the surface, gases start to bubble upwards through the magma and build pressure against the crust. When the roof of a volcano is blown, the magma is no longer under the pressure that keeps these volatiles liquid, so they turn to gas and expand greatly, sometimes up to 1600 times their original volume (Hazlett 2013). The size of the eruption depends on the volume of magma being purged from the chamber, the amount of volatiles in the magma, and the viscosity of the magma. An eruption can be quantified and put on a scale called the VEI scale, or volcanic explosivity index. The VEI of a volcano is based on the volume of erupted tephra (ash fallen from the air) and eruption plume height. Any eruption that releases more than 1000 km3 of ejecta earns a VEI above 8 and is considered a "super eruption." The largest recorded eruption is credited with a 9.1 VEI, while the Bishop Tuff eruption is ranked as an 8.1 on the VEI scale (Mason 2004).
Volcanism in the Long Valley area began roughly 3.2 million years ago. Due to the extensional forces of Basin and Range-related movement, basaltic magma found its way to the surface through vents spanning a range of over 4000 km2 (Hill, Bailey 1985). The magma of these early eruptions was chemically different from that of the Long Valley eruption. The first evidence of high-silica magma was found near Glass Mountain, where it began leaking to the surface through ring-fractures roughly 2.1 million years ago. The ring-fractures were caused by the large magma chamber coming closer to the surface, bulging it outwards. Using K-Ar dating of rhyolitic rocks on Glass Mountain, it is believed that the mountain was built by eruptions spanning over roughly 1 million years. The rhyolite of this area has provided clues to the nature of the magma just before the Bishop Tuff eruption. Containing 77% silica, the magma was very volatile-rich and highly evolved (Hill, Bailey 1985). While the initial source of the magma body is not quite clear, it is believed that fractional crystallization of the basaltic magma and mixing with continental lithosphere may be the cause of the high silica content (Knesel, Davidson 1997). Using seismic data, mapping of the magma chamber shows that the top of the chamber lies 6-7 km under the surface. The chamber also features a section of magma protruding to within 4.5 km of the surface near the middle of the chamber, and possible dykes extending to within 5-6 km of the surface in the south of the chamber. The total volume of magma in the chamber is estimated to be 500-1000 km3 (Hill, Bailey 1985).
Bishop Tuff Eruption
760,000 years ago, the roof of the magma chamber was blown and erupted in a plinian event. Almost immediately after the partial emptying of the magma chamber subsidence of the area occurred, creating the caldera we see today. The subsidence occurred along ring faults, most of which are buried today. The present size of the caldera is larger than its initial size as erosion has taken effect on the boundaries (Bailey 1976). A plinian eruption is characterized by a large explosion and a huge column of ash and pumice shot upwards, sometimes up to 40 km. As gravity begins to take effect, the ejecta falls to the earth as a pyroclastic flow, moving up to hundreds of meters a second. These flows deposit in a well-documented pattern, starting with a bed of well-sorted pumice under cross-beds of pyroclastic surge flows. This is followed by poorly sorted pyroclastic deposits containing pumice, ash, and lithic fragments. On top of this is a cap of unstratified ash (Hazlett 2010). The eruption released an estimated 500 km3 of ash, reaching as far as Nebraska (Bailey 1976). The ash, known as Bishop Tuff, spread radially through the area, being deposited as deep as several hundred meters in proximal locations (Bailey 1976). The most apparent view of the ash flow is the Volcanic Tableland, which shows sharp contrast between the ash and Sierran lithic material. The estimated coverage area of the tuff is around 1100 km2 , but a majority of the ash stayed within the caldera. Long since covered by postcaldera rhyolite and sediment of surrounding areas, tuff deposits within the caldera are estimated at 1000-1500 m thick. Up to 2/3 of the total ash released in the eruption stayed in the caldera (Bailey 1976).
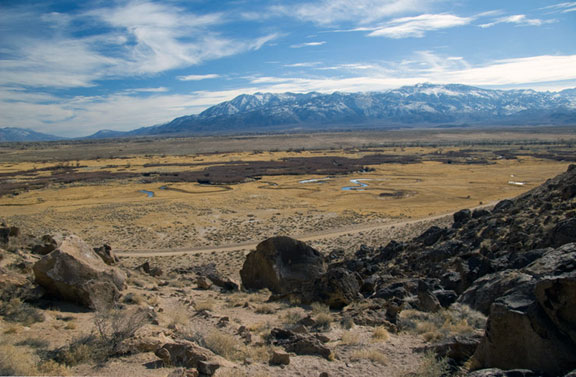
Standing on the Volcanic Tableland, the Sierra Nevada Mountains lie across Owens River flood plain. Used from Mountainproject.com.
Welded Tuff
The tuff of the Long Valley eruption is special in that it exists as a "welded" material in some areas. As the hot ash accumulated through air fall and ground flows, it began to cool and compress into a single, massive structure. According to Smith (1960), these units of solid deposits are known as simple cooling units. Throughout the cooling units, degree of welding varies as depth of the unit changes. Basal and capping levels of the unit have low degree of cementation, as the cool ground and air prevents strong welding. The strongest welding occurs in the lower half of the unit, as that is where heat is retained the longest (Hildreth 1979). While single units are all composed of uniform mineralogy, slight differences in chemical composition, first observed at Owens River Gorge in the 1960’s, have helped prompt research to define the different cooling units and create an eruption sequence. According to Hildreth (1979), there are eight cooling units that can be defined. Study of these units and their geographic locations can provide insight to how long it took the tuff to cool, what area of the caldera the ash came from, and direction and timeline of ash flow. Interpretations based on cooling unit observations are still debated to this day. Hildreth (1979) and Hildreth, Wilson (2006) believes that based on relationships between cooling temperature and composition of various units, the eruption would have been nearly continuous lasting for only about 6 days. Others, such as Snow and Yund (1998) have reached the conclusion that in some areas, hiatus between depositions may have been up to 2 years. In other places, breaks in ash deposition may have only been up to 40 days (Hildreth, Mahood 1986).
The Magma Chamber
It is believed however that the eruption occurred in a ring-fracture style, featuring multiple vents and changing vent location throughout the eruption, rather than erupting through a single vent (Hildreth, Mahood 1986). This supports the claim that there are varying cooling times for deposits in different locations. What can be agreed upon is that the initial plinian event occurred in the southeast area of the caldera (Hildreth, Mahood 1986). This is supported by absence of lithic fragment that are found in the northern part of the caldera and the fact that the tuff to the south of the caldera is thick and highly welded but thin and non-welded to the north. Another supporting factor is that the southern part of the caldera features faulting that may have acted as a pathway for the magma to collect there in the first place (Hildreth, Mahood 1986). After the initial plinian event in the southeast of the caldera, the vent location changed as the caldera "unzipped," in the words of Holohan, E.P., Troll, V.R., De Vries, B.V.W., Walsh, J.J., and Walter, T.R. (2008). Their research has shown that because the caldera is in a highly elliptical shape, when the caldera began to sag and decompress the strain along the roof of the chamber caused vent migration along the short axis. Vent migration is supported by the physical modeling of the group, as well as subtle differences in mineralogy of outcrops based on what vent the ash was released from (Holohan, E.P., Troll, V.R., De Vries, B.V.W., Walsh, J.J., and Walter, T.R. 2008).
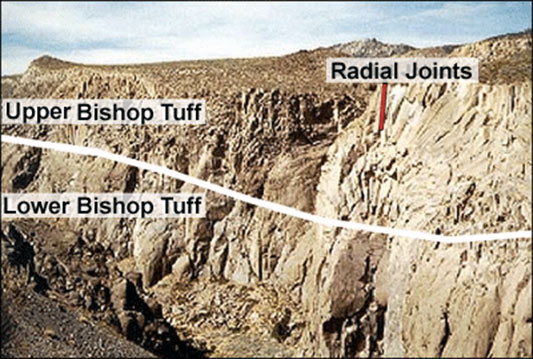
Multiple cooling units at Owens River Gorge. Also shows joints formed by steam vents. Used from http://www.dpc.ucar.edu/earthscopeVoyager/JVV_Jr/didyouknow/images/volTuffsPix.gif
What is also known about the eruption is that all tephra originated from a single magma chamber (Hildreth, Wilson 2006). Their conclusions state that based on the mineralogy of the cooling units and the types of pumice found, the eruption was fueled by a single magma body with coolest temperatures, highest in gas content, and most differentiated at the roof. They also found that it is likely that shortly before eruption, the high-silica rhyolitic magma was injected with lower silica dacitic magma. These facts are supported by a single caldera rather than multiple, absence of contradicting ash deposits, mostly uniform mineralogy, and presence of sparse dark and swirly pumice found sporadically throughout the various cooling units.
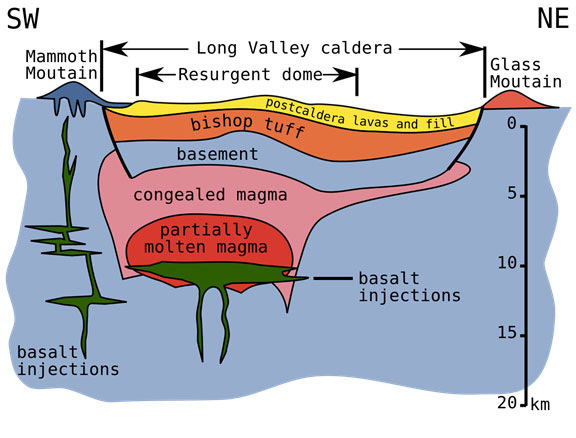
Current cross section of the Long Valley Caldera
"http://upload.wikimedia.org/wikipedia/commons/thumb/3/3d/Long_Valley_Caldera_cross_section.svg/1280px-Long_Valley_Caldera_cross_section.svg.png
Mineralogy of the Tuff
One thing that has been observed in the Bishop Tuff is the gradual change in mineralogy as the eruption endured. Some of the minerals present in the tuff are Fe-Ti oxides, quartz, sanidine, plagioclase, biotite, and small amounts of pyroxenes, allanite, and zircon (Hildreth 1979). Quartz and sanidine are most abundant, mostly in similar proportions, with plagioclase the next abundant. Together these crystals make up 98-99% of total crystals found in the Bishop Tuff (Hildreth, Wilson 2006). According to the abundance of these minerals in the various cooling units, geologists are able to tell when the cooling unit was deposited in relation to other units. The initial plinian explosion, which distributed ash the farthest, was small and contained few phenocrysts. After this blast, similarities in composition indicate that ash flows made their way towards the east, southeast, and southwest. These were the hottest flows and contained some less common minerals such as augite and hypersthene (Hildreth 1979). As the venting migrated more northward, ash originating from deeper magma began to be released. The deposits associated with these vents include more abundant levels of pyroxenes and pyrrhotite. These minerals are associated with higher temperature conditions (Hildreth 1979). It can be interpreted that the magma at the roof of the chamber was the coolest at the time of eruption, containing the fewest phenocrysts. As the venting migrated due to ring fractures, the caldera began to collapse, forcing deeper, hotter magma out of the vents (Hildreth 1979).
Conclusion
The Bishop tuff eruption, which created a 450 km2 caldera that we still see today, released an outstanding amount of tephra, estimated to be in excess of 500 km3, coating much of the western United States. After an initial plinian event, ash flows blanketed proximal and medial locations in an overall rhyolitic ash, measuring approximately 77% silica. These ash flows accumulated quickly, in some places as high as 150 m. In these places, the hot ash condensed and solidified, creating what we now observe as welded tuff. Appearance of multiple cooling units tells geologists that the ash flows came in waves with little hiatus between them. Slight variations in mineralogy of these cooling units suggest that there were multiple vents from which ash flowed rather than one single vent. These migrating vents all tapped from a single magma chamber, which is of unknown origin other than it is probably related to Basin and Range extensional forces. There is still much we don’t know about one of the largest eruptions in recent history, making it an ever-intriguing area of study for novice and seasoned geologists alike.
Works Cited
Bailey, R.A. (1976). Volcanism, Structure, and Geochronology of the Long Valley Caldera, Mono County, California. Journal of Geophysical Research 81(5): 725-744.
Davidson, K.M. K.A. J.P. (1997). The Origin and Evolution of Large-Volume Silicic Magma Systems:Long Valley Caldera. International Geology Review 39(11): 1033-1052.
Hazlett, L.A. (2013). Volcanoes:Global Perspectives, Blackwell Pubishing.
Hill, M. (2006). Geology of the Sierra Nevada. Las Angeles, University of California Press.
Mahood, W.H.G. (1986). Ring-fracture eruption of the Bishop Tuff. Geologcal Society of America Bulletin 97(4): 396-403.
Mason, B.G. (2004). The Size and Frequency of the Largest Explosive Eruptions on Earth. Bulletin of Volcanology 66(8): 735-748.
Wilson, W.H.C.J.N. (2006). Compositional Zoning of the Bishop Tuff. Journal of Petrology 48(5): 951-999.