Abstract
The Long Valley Caldera is the site of one of the largest eruptions ever to occur in geologic history. After expelling much of its magma, the region did not stop being volcanically active. Numerous eruptions have taken place in this region over the past 760,000 years. The caldera has recently shown increasing volcanic activity, concerning many that future eruptions could be imminent. New methods have been developed to carefully observe and analyze volcanoes to be able to better understand the activity currently seen and to predict future eruptions. Uplifting, hot springs, fumaroles, earthquakes, and various other features all point towards magma as their cause. Basaltic magma intrusions have caused much of the activity, both recent and throughout the time period following the Long Valley eruption, as the magma chambers become replenished and more recently as the magma affects the surrounding rocks. The tectonic setting causes the volcanic aspects of this region.
Introduction
Forms of Volcanic Monitoring
Volcano monitoring takes place around the world in places that have been volcanically active in the past in an effort to predict when a future eruption is likely to go off. This science involves watching for any changes in a volcano that could indicate an eruption is imminent. This process has saved thousands of lives and prevented terrible tragedies in some cases. One such case where tragedy was avoided is the 1994 eruption at the Rabaul Caldera in Papua New Guinea. For a fifteen year period, between 1971 and 1985, the amount of uplift at the caldera was approximately 2 m, but in one day, the amount of uplift in some areas of the caldera was as much as 6 m (McKee 1). This rapid change in the rate of uplift was a sure sign of increased volcanic activity for geologists, who warned the people in time for a complete evacuation. Of the 50,000 inhabitants, only five died as a result of the eruption ("Rabaul" 3).
There are multiple ways of monitoring a volcano, all of which can provide critical information about its activity. One method of monitoring a volcano is GPS. GPS can be used to show deformation of a landform over time by comparing the coordinates for a location over time. Normal GPS devices have an accuracy within a few meters, with error being generated from a variety of sources, like clock error, atmospheric interference, or indirect signals (Bartel). Various methods of obtaining GPS coordinates have been developed to minimize error and achieve precision to a few centimeters or less ("How We Monitor Volcanoes," 4). Differential GPS uses a base station and calculates the coordinates relative to the base for greater precision (Bartel). The most precise method currently available is done by using multiple GPS units across a volcano or other feature, and recording the coordinates over an eight hour period, or longer to eliminate atmospheric interferences ("How We Monitor Volcanoes," 4).
Other methods of measuring volcanic activity include leveling, tiltmeters, InSAR, and monitoring gas emissions. Leveling measures the difference in elevation from one location to another, and observes the changes over time at these set locations to measure deformation. Tiltmeters measure changes in slope with an accuracy of .00006 degrees ("How We Monitor Volcanoes," 5). InSAR is a satellite imagery technique that shows deformation by comparing satellite images over time (8). Gas monitoring involves measuring the rate of gas emissions from soil, continuous monitoring of a specific gas from a vent over time, or determining the types of gases and measuring the concentrations of them at one time (9).
Perhaps the most important part of volcano monitoring is looking at seismic activity. Prior to many eruptions, there is an increase in earthquake activity below the volcano. For example before the 1980 Mount St. Helens eruption, between the end of March and to the middle of May there were over 10,000 earthquakes centered at a depth of around 2.5 km beneath the volcano (Tilling, 1). Another precursor to many eruptions is a volcanic tremor. Volcanic tremors are similar to earthquakes in that they generate frequency waves, but these waves tend to last much longer—several minutes and sometimes even days—while most other earthquakes are short in comparison ("How We Monitor Volcanoes," 2). Volcanic tremors began occurring about a month and a half before the Mount St. Helens eruption, and they also have commonly been observed at volcanoes worldwide (Tilling, 1).
Long Valley and Mono Basin Geologic History:
These methods of monitoring volcanoes have been used at the Long Valley Caldera since 1980, when seismic unrest prompted an increased understanding of the volcanic processes in this area ("California Volcano Observatory," 1). The Long Valley Caldera is a large 17 km by 32 km depression in the Eastern California region formed by a massive eruption 760,000 years ago ("Geologic History," 1). Calderas are unique from other types of volcanic craters in that they form by the ground actually sinking after the eruption; when the high volume of magma is ejected, the overlying earth falls in on the depleted magma chamber. The Long Valley eruption spewed out approximately 600 km3 of magma, almost 2000 times as much magma as the Mount St. Helens eruption 35 years ago ("Volcanic History," 1).
The volcanic processes in the Long Valley area did not cease after the cataclysmic eruption 760,000 years ago. During the 100,000 years after the caldera–forming eruption, several small eruptions took place in the caldera zone, followed by the formation of the resurgent dome ("Geologic Map," 2). The next major sequence of eruptions occurred in the caldera moat, which surrounds the resurgent dome. These three eruption sequences took place every 200,000 years beginning approximately 500,000 years ago, and finishing 100,000 years ago (2). The eruptions that formed Mammoth Mountain and the first Mono-Inyo chain eruption occurred after this along a formerly active fracture system (Tierney, 55). The oldest eruptions along the Mono-Inyo chain have been covered up by the more recent–less than 40,000 year–old–eruptions, which formed the craters now visible today (55). The most recent eruption in this area occurred approximately 220 years ago on Paoha Island in Mono Lake ("Geologic Map," 4).
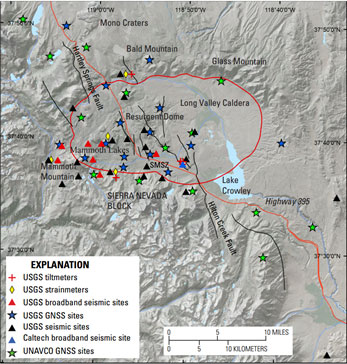
Figure 1. This map of the Long valley Caldera and the surrounding regions shows the location of various volcanic and seismic monitoring devices in the area (Wilkinson 2).
Modern-day Concerns and Monitoring in the Long Valley Region
After its extensive volcanic history, many people are concerned over the likelihood of future eruptions in the Long Valley-Mono Basin area. Extensive monitoring began in the area after six earthquakes with magnitudes 5.7 to 6.2 occurred within three days of each other (Ryall and Ryall, 1432). High levels of volcanic alert were established in the Mammoth area after these earthquake, causing severe economic problems and a tourist crisis for the people of Mammoth (Wheeler, 45). Figure 1 shows the vast number of devices, including global network satellite system (GNSS) devices, tiltmeters, and various devices to monitor seismic unrest. InSAR and leveling have also been used to observe changes in this area and gas monitoring have been used in this area as well, focusing especially on the Resurgent Dome (Tizanni, et al., 63). A large quantity of data has been collected for this area to see the changes overtime as accurately as possible. The Mammoth scare along with the vast number of devices in the area and the extensive data collecting show how concerned the USGS and other geological groups are with regards to the volcanic processes in the Long Valley, indicating that observation for future volcanic eruptions is critical for this area.
Observations
Water Features
The Long Valley Caldera and Mono Basin are known for many of the water features located around the area. Hot springs are located in the caldera at various locations, including the aptly named Hot Springs, and in the Mono Basin, hot springs can be found on Paoha and around Black Point ("Geologic Map" 7). Long Valley also is a supplier of geothermal energy, which is harnessed at the Casa Diablo geothermal plant; water reaches temperatures of around 160°C when it makes its way towards the surface (Sass and Priest, 185). The water stored deep in aquifers reaches even higher temperatures, with 220°C being the highest observed temperature for the region, although higher temperatures are likely to exist for the aquifers down below ("Geologic Map," 7).
Resurgent Dome Activity
After the hydrothermal solution is formed, it then flows through the path of least resistance through porThe Resurgent Dome has been undergoing significant changes since observation of it began in 1980. Data from InSAR, leveling, and GPS all show that the Resurgent Dome has been trending such that it is rising and expanding (Tizanni, et al., 64). In the ten year period from 1989 to 1999, the Resurgent Dome experienced 80 cm of uplift ("Eruptive History," 31). The rate of uplift has not been continuous however. Sometimes the dome rises at a rapid 20 cm per year, and at other times the dome seems barely to be rising at all (43). Also, the rate of uplift has not been uniform on every part of the Resurgent Dome. In particular, in the southern edge of the dome, the amount of uplift varies notably (32).
The changes in gravity on the Resurgent Dome have been studied over time to see slight changes that indicate changes in the mass below the dome (Tizanni, et al., 65). The measured changes in gravity were altered to minimize the effects of water table levels and other outside factors on the data. The data showed that the mass of the area increased–something was added into the crust (66). The density of this material was determined to be between 1200 and 2300 kg/m3 (64).
Seismic activity in the region can easily be monitored and recorded with seismographs. Countless seismic events have been observed since the six earthquakes in 1980 that showed that unrest in the area. Beneath the south moat, earthquake swarms–large numbers of earthquakes occurring in a short time period–have occurred from time to time since 1980 ("Eruptive History," 2). Also, times of heightened uplifting for the Resurgent Dome are associated with strong earthquakes (Ryall and Ryall, 1433).
Rock Types of Paoha
In the Mono Basin, Paoha is an island made partly of sedimentary rock and partly of volcanic rock. The sedimentary rock was deposited into a lake bed and exposed around 300 years ago (Tierney, 54). The volcanic rock is from the eruption on Paoha that occurred 250 years ago (54). In addition to the hot springs on this island, the volcanic island also has fumaroles, which emit gas into the air ("Geologic Map," 7).
Gaseous Observations
The greatest emission of gases in this area is occurring at Mammoth Mountain. At Mammoth, several acres of trees mysteriously began dying in the 1990s, but a few years later the cause was determined to be carbon dioxide poisoning (Wheeler, 47). So much carbon dioxide was in the soil that it essentially drowned the trees of the other gases they needed to take in from the soil. The amount of carbon dioxide emissions have been estimated to be approximately 500 tons per day around Mammoth (48). Gas pockets have also been noted to exist in the Mono Basin, found during the creation of the aqueduct system underground which diverts water from streams that feed Mono Lake (Tierney, 51).
Historical Observations and Eruption Sequences
Additional observations about this region involve the geologic history. The Mono-Inyo chain has been very active over the past 5,000 years. There has been an eruption every 250 to 700 years in this chain in this time period (Hill, 24). Many of these eruptions have been explosive, such as the phreatic explosions that formed the Inyo domes a little over 600 years ago ("Geologic Map," 4). Figure 2 shows that the vast majority of the eruptions in the region in the past 5,000 years have indeed been explosive eruptions. The eruptions that occur in this chain tend to follow a pattern. First, a powerful eruption releases much of the pressurized gases (Tierney, 52). Phreatic eruptions like what formed the Inyo Craters can also take place at this time ("Geologic Map," 4). Next, rhyolitic lava effuses out, creating the dome structures seen along the chain (Tierney, 52). The thick lava then flows out to form a coulee structure (53).
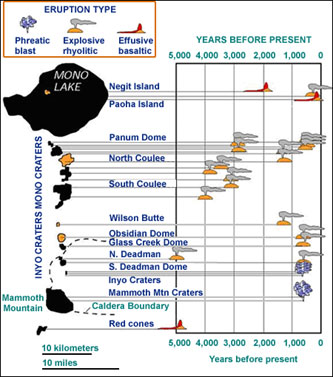
Figure 2. This figure shows the eruptions that have taken place in the Long Valley region over the past 5,000 years along with the type of eruption ("Volcanic History," 3).
Tectonic Setting
One final observation involves the tectonic setting of the Long Valley Caldera and Mono Basin region. This area is located in the Basin and Range Province which is being created by continental extension of the western North American Plate as it slides past the Pacific Plate (Hill, 22). As continental extension occurs, the crust becomes thinner and often breaks, in faults, fissures, or joints (22).
Interpretations
Cause of the Features and Recent Activity
All the features aforementioned hot springs, fumaroles, uplifting of the Resurgent Dome are caused by current volcanic activity in the Long Valley Caldera and Mono Basin region. The hot springs and the ability to use geothermal power are created by magma heating the aquifers in the crust and causing the now-buoyant liquid to rise ("Geologic Map," 7). Fumaroles are formed by gases being released from magma, similar to how carbon dioxide bubbles slowly seep out of a soda over time (Wheeler, 42).
The Resurgent Dome is being uplifted due to changes taking place in deep in the crust. The gravity study of the Long Valley Caldera showed that approximately a volume increase below the surface would have to cause the uplift, and the density and setting implied that the most likely explanation is a basaltic intrusion mixing with hydrothermal fluids (Tizanni, et al., 66). Magma filling in the magma chamber is causing this uplift. However, the variation between the southern part of the Resurgent Dome and the rest of the dome means that the situation is not quite as simple as one magma chamber being filed and causing the uplift. Two magma chambers exist under the Resurgent Dome: one is approximately 6 km deep, and the one beneath the south end is smaller and approximately 10 km deep (Hill, 32).
The Resurgent Dome is not the only place to have undergone uplifting. The island of Paoha was once under Mono Lake’s surface, but volcanic processes brought this lake to the surface. Like the Resurgent Dome, magma intrusion forced the lake bed to rise in this area (Tierney, 54).
The earthquake swarms in the region are also the result of magmatic activity. As magma gets added to a chamber, the stress on the surrounding rocks increases. The stress accumulates until the surrounding rocks crack, creating a series of earthquakes ("How We Monitor Volcanoes," 2). Fluids and gases moving through by and through cracks can generate shaking in the cracks which also makes seismic waves (2).
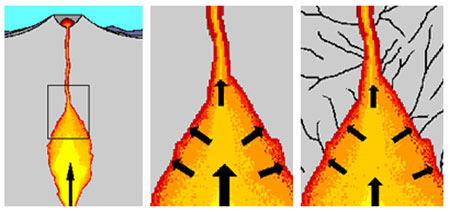
Figure 3. Magma adds stress on the surrounding rocks and causes fracturing ("How We Monitor Volcanoes," 2).
The Current Magma Chamber and Future Eruptions
The Long Valley Eruption was the result of erupting one huge magma chamber, but such a chamber no longer exists beneath Long Valley. Instead, now there are multiple, smaller chambers under the area (Wheeler, 46). So, a cataclysmic eruption like what occurred 760,000 years ago is incredibly unlikely (46). Even the smaller eruptions are not very likely for any given year. Based on the long-term eruption sequence along the Mono-Inyo chain of an eruption every 250 to 700 years, there is a .05% chance of an eruption per year (Hill, 24). However based on past eruption sequences, powerful rhyolitic explosions followed by effusive rhyolitic eruptions are likely the type that will occur in the region (Tierney, 56).
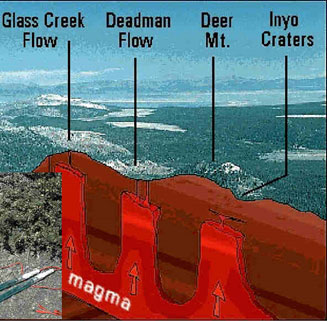
Figure 4. Separate magma chambers beneath the caldera formed the various features seen here ("Environmental Geophysics," 1).
The Effect of Plate Tectonics
The final question about this region is why magma is present there in the first place to cause all these other processes. The volcanic processes are a direct result of the tectonic processes of the western North American Plate. Basalt injections into the magma chamber are caused by Basin and Range tectonics as the continental stretching leaves space that the magma goes into to fill (Ryall and Ryall, 1433). Continental stretching has been going on for a long time, and similarly basaltic injections have been occurring multiple times since the Long Valley eruption to fuel the multiple eruptions that have taken place over the past thousands of years ("Geologic Map," 2). The locations of these eruptions are also connected to the tectonic processes. Magma needs a pathway to the surface, the faults, joints, and fissures created by the tectonic environment (Tierney, 53).
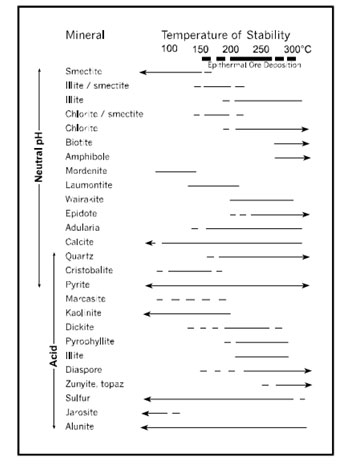
Figure 3. This diagram depicts the various temperatures at which common hydrothermal minerals in epithermal deposits achieve stability. In acid-sulfate alteration deposits, which are high-sulfidation systems, the main gangue mineral is quartz. In adularia-sericite alteration deposits, which are low-sulfidation systems, the main gangue minerals are calcite, adularia and quartz [Hedenquist, 1995].
Conclusion
The Long Valley region is still a highly active volcanic area even though no eruptions have taken place in our lifetime. Evidence of its activity can be seen in the popular hot springs, the trees killed by carbon dioxide, and the uplifting. An eruption is very likely to occur at some point in this region based on the volcanic history and the current processes. The forces that caused the initial magma intrusions in the Long Valley region are still active today, and they are still causing magma to be intruded into this region.
Towns like Mammoth Lakes and Lee Vining are well within the vicinity of the area that could be affected by volcanic eruptions in this region. The potential for an explosive volcanic eruption and the effects it would have on the population centers in the area have kept geologists extra cautious when it comes to this area. Monitoring is extremely thorough and response plans have been established, including a backup escape road on Mammoth Mountain (Wheeler 46). Scientists tread carefully before sounding any form of alert because of public backlash that has occurred after the Mammoth 1980 alert (46). Despite their worry about public issues, the primary concern of the USGS and the geologists studying this area is the safety of the people, so careful monitoring with necessary warnings will continue into the future to monitor the giant today.
Works Cited
Bailey, R.A. Eruptive History and Chemical Evolution of the Precaldera and Postcaldera Basalt-Dacite Sequences, Long Valley, California: Implications for Magma Sources, Current Seismic Unrest, and Future Volcanism. USGS. US Department of the Interior. 2004. Web. 6 June 2015.
Bailey, R.A. Geologic Map of Long Valley Caldera, Mono-Inyo Craters Volcanic Chain, and Vicinity, Eastern California. USGS. US Department of the Interior. 1989. Web. 12 June 2015.
Bartel, B. GEOL G-190, Indiana University. Sierra Nevada Aquatic Research Lab, Mono County, California. 21 May 2015. Lecture.
California Volcano Obseratory. USGS. US Department of the Interior. 1 June 2015. Web. 12 June 2015.
Environmental Geophysics/Geoelectric. University of Leipzig. University of Leipzig. 2015. Web. 14 June 2015.
Geologic history of the Long Valley, Mono Basin region. USGS. US Department of the Interior. 6 Feb. 2012. Web. 8 June 2015.
Hill, D.P., et al. Response Plan for Volcano Hazards in the Long Valley Caldera and Mono Craters Region. USGS. US Department of the Interior. 2002. Web. 6 June 2015.
How We Monitor Volcanoes. USGS. US Department of the Interior. 13 Aug. 2008. Web. 9 June 2015.
McKee, C.O. Rabaul Caldera, New Britain Island, Papua New Guinea. Oregon State University. Volcanological Survey of Indonesia. 1997. Web. 8 June 2015.
Rabaul. Oregon State University. Volcano World. n. d. Web. 9 June 2015.
Ryall, A., and Ryall, F. Spasmodic Tremor and Possible Magma Injection in the Long Valley Caldera, Eastern California. Science Vol. 219, No. 4591 (25 Mar. 2015). JSTOR. Web. 12 June 2015.
Sass, J., and Priest S. Geothermal California. Geothermal. US Geothermal Development. Oct. 2002. Web. 11 June 2015.
Tierney, T. Geology of the Mono Basin. Mono County: Kutsavi Press, 1995. Print.
Tilling, B. History–Mount St. Helens. MountStHelens.com. Natures Discovery LLC. 2012. Web. 13 June 2015.
Tizanni, P., et al. Uplift and magma intrusion at Long Valley caldera from InSAR and gravity measurements. Geology. Geological Society of America. Jan. 2009. Web. 9 June 2015.
Volcanic History of Long Valley Caldera. EarthScope Voyager Did You Knows. EarthScope Voyager. n. d. web. 8 June 2015.
Wheeler, M. When Magma’s on the Move. Smithsonian. Smithsonian Mag., 1 Feb. 2000. Web. 6 June 2015.
Wilkinson, S., et al. Long Valley Caldera 203 through 2014: Overview of Low Level Unrest in the Past Decade. USGS. US Department of the Interior. 2014. Web. 12 June 2015.