Abstract
GPS data shows that the previously seasonal rate of uplift of the Sierra Nevada range in eastern California has increased during the current drought. This paper begins by exploring the background of the geological setting, such as the formation and composition of the range, and comparing geological behavior under normal and drought climate conditions, so that the role of the drought in the uplift of the Sierras and the potential for human impact on tectonic environments may be elucidated.
Introduction
There is evidence that the Sierra Nevada was uplifting steadily, but seasonally. As the heavy snowpack that forms in the winter melts each spring, the weight of the snow lessens and the compressional forces on the mountain range are reduced; allowing for a more rapid uplift. With the current extreme drought that California is experiencing, the snowpack on top of the Sierras has been significantly less massive than its typical annual averages. With less weight on the tops of the mountains, it appears that they are uplifting more rapidly as a result, having an impact on the surrounding geological environment. The importance of this is that if the drought is a result of human-caused climate change, then human action has the capability to have a major impact on the tectonic environment of the planet.
Geological Setting
Quick Geological History of the Sierra Nevada
The North American tectonic plate did not originally include most of what is today California. 400 million years ago, a subduction zone formed between two seafloor plates that were adjacent to the North American plate. This collision of plates and the upwelling of magma from the dissolution of the subducted plate formed arcs of volcanic islands in the ocean. These chains of volcanic islands then collided periodically with the continent, adding their sediment and some of the seafloor to its western edge. The rifting that caused the breakup of the Pangaea supercontinent 215 million years ago changed this tectonic environment, causing the Fallaron seafloor plate to subduct beneath the continental North American plate. The magma formed from the upwelling of the subducted seafloor plate caused the genesis of an inland volcanic chain: the Sierran Arc volcanoes. Sierran Arc volcanoes were active until another major change in the global tectonic environment "turned off" Sierran Arc volcanism 70 million years ago (Tierney, 2000).
The water and volatiles present in the subducting Fallaron seafloor plate were superheated by the mantle and rose upward out of the downward-moving plate and into the crustal plate above, heating the rock to the point of melting, forming pockets of magma. These either cooled very slowly into plutons of intrusive granite or were erupted by the Sierran Arc volcanoes (Hill, 2006). These plutons of granitic rock now form the backbone of the Sierra Nevada range, and are collectively known as the Sierra Nevada Batholith.
The Fallaron plate eventually disappeared beneath the continent, exposing the underside of the crust to the mantle once more. Deformation occurred as the crust was heated, causing the thinning and stretching that created the current Basin and Range region to the east of the Sierra Nevada. Some researchers doubt that the Sierra Nevada’s uplift was influenced by this same event, however it is important to the Sierra region as it provides some geological and climactic context.
Theories of the Orogeny of the Sierra Nevada
There is contention in the field of geology about the timing and mechanisms of the uplift and tilt of the Sierra Nevada. According to the summary provided by Christopher D. Henry in his 2009 paper Uplift of the Sierra Nevada, California, there are a few major uplift events that cause this controversy. The first being the original theory: that uplift occurred in the late Cenozoic due to the entire fault-block uplifting on the steeper eastern side and tilting the whole block to the west. The second and more modern theory is that the original uplift occurred much earlier, in the late Mesozoic or early Cenozoic. Henry claims that data from two separate papers, Mulch et al., 2006 and Cassel et al., 2009, support the idea that uplift occurred "probably as a result of crustal thickening related to contraction and voluminous batholithic magmatism." (Henry, 2009). It seems that many geologists agree on these uplift events but there is contention as to the mechanisms that cause them.
In his paper published in 1991, J.R. Unruh used the degree of tilt found in Tertiary strata on the eastern flank of the Great Valley in California to determine the timing and the rate at which uplift and the tilting on the Sierra block occurred (Unruh, 1991). In this paper, Unruh cites paleobarometric analysis on plutons of rock in the Sierra performed by other researchers that supports that significant uplift took place between the early Cretaceous and the Eocene. His proposed (but untested) mechanism for the late Cenozoic uplift of the Sierra is the thinning of the Sub-Sierran mantle lithosphere. He suggests that this uplift is a part of a larger mantle-thinning event that effected many parts of the Cordillera plateau, parts of which exhibit the high elevation and thin crust that support this claim (Unruh, 1991).
Figure 1. Schematic block diagram illustrating the simple kinematic model for uplift of the Sierra Nevada. The Sierra Nevada has been uplifted and tilted westward, forming a distinctly asymmetric profile. The northwest-southeast-striking late Cenozoic tilt axis is in the eastern Great Valley. As a consequence of the uplift, Cenozoic
Eric E. Small and Robert S. Anderson demonstrate in their 1995 paper that the majority of the 2,000 meter uplift seen in the Sierras in the late Cenozoic could have been largely an effect of isostatic adjustment to the erosion of the already high Sierra. Isostasy is the major driver in this argument. This principle states that if a mountain peak erodes slightly, it will rise in response to the reduced load on its surface (Tarbuck and Lutgens). They argue that the amount of tectonic forcing that others previously attributed to this event is not necessary in their model of uplift due to geomorphic forcing (Small et al., 1995).
Much of the recent evidence for the earlier uplift of the Sierra Nevada comes from chemical analysis of isotopic deposits from the rain shadow on the eastern side of the Sierra. Using this method, researchers can determine paleoelevation of the landscape and create a timeline of elevation. Still other researchers (Zandt et al., 2004) implicate the removal of the batholith’s ultramafic root by convection in the mantle, and then isostatic adjustment as a potential reason for elevation change in the Sierra.
The Sierras have an incredibly complicated history of uplift that is still evolving as the mountains are themselves dynamic and the research tools available to scientists become more specialized and sophisticated. With time and continued research their story will continue to be clarified.
Water in the Sierra
Typical Hydrology of the Region
Up to sixty percent of the precipitation in California comes from seasonal rains or snowfall in the Sierra Nevada and the southern Cascade Range (Sierra Nevada Conservancy, 2011). The majority of the precipitation in California blows in as water vapor from the Pacific Ocean. As the vapor-bearing air goes farther inland and reaches the mountains, it gains altitude and cools, losing energy and its capacity to retain gaseous water. When the wind loses the energy required to carry the water vapor, the water then falls as precipitation; either rain or snow depending on conditions and the elevation at which the precipitation falls. In warmer spring months, the snowpack that has developed as a result of winter storms begins to melt and release water as run off into the valleys below the ranges.
According to the report recently released by the California Department of Water Resources, the state receives 75% of its annual rainfall between November and March, and half its annual rainfall from December to February. The majority of moisture-bearing winter storms are a specific kind called atmospheric river storms which are "storms fueled by narrow regions in the atmosphere that transport a concentrated stream of water vapor across the Pacific to the West Coast" (California Dept. of Water Resources, p. 10, 2015). Summers are drier due to a high pressure system that forms off the coast, deflecting weather systems like the atmospheric river storms from the Pacific Ocean away from the coast.
Due to this highly variable and storm-dependent water system, there are relatively few permanent aboveground rivers and lakes in California. Those bodies of water that do exist on a permanent basis are diverted extensively for human use, whether that is for agriculture, energy, or domestic use. The amount of surface water present in California is insufficient to meet the demands of the many water-users in the state; therefore, they turn to groundwater.
According to the report prepared by the California Water Resources Department on the current drought, under normal hydrologic conditions the alluvial basins where groundwater accumulates provide up to 40% of the water used for agriculture and by urban water users. During a drought, however, this percentage increases as surface water becomes less available (California Dept. of Water Resources, p. 15-17, 2015). Large scale extraction of groundwater can lead to ground subsidence, and this phenomenon has been monitored by the US Geological Survey for roughly 80 years. Land subsidence has occurred during past droughts, periods of high groundwater extraction, but had recharged in the wet years since. However, due to new environmental laws that limit the amount of water that can be diverted from surface water rivers and lakes, the amount of groundwater extraction has been increasing steadily since the 1990s and subsidence of the floor of the Great Valley is visible once more (California Dept. of Water Resources, p. 37, 2015).
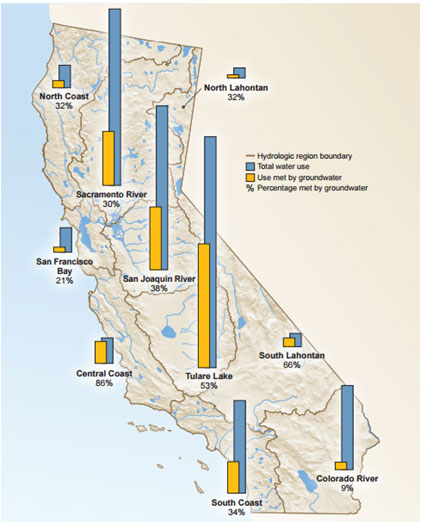
Figure 2. Groundwater contribution to total water use by hydrologic region. California Dept. of Water Resources, 2015.
Hydrology of the Current Drought
California is currently experiencing an incredibly acute drought. The years 2012-2014 have been the three driest cumulatively for the state ever with 2015 looking to continue that stretch after another winter of low precipitation. Along with the driest conditions California has experienced in recorded history, the past three years have also seen a record-breaking increase in temperatures for the state. This current drought also marks only the second time that a drought has ever been declared on a state level. However, the lack of previously declared state-wide droughts does not necessarily indicate that California has not had severe droughts before, but that California lacks a unified set of conditions and benchmarks that indicates to state and regional government that one is occurring (California Dept. of Water Resources, p. 4, 2015).
Effects of Modern Water Situation on the Sierras
There have been several recent studies about the effects of the loss of water from the surface and sub-surface on the behavior of the crust in California and the rest of the western United States. Amos et al., 2014 and Borsa et al., 2014 both used the extant networks of GPS stations across the west (and to a lesser extent satellite gravimetry, or GRACE) to measure vertical movements of the crust in response to water levels and precipitation patterns.
The amount of water in the Sierra Nevada varies cyclically with the seasons. A wet winter produces heavy snows that accumulate in the mountains in a snowpack and add their mass to that of the mountains. This affects the mountains, slowing their uplift slightly, then accelerating the uplift as the snow melts and runs down in the spring. The drought changes these weather patterns, and these studies took place to determine whether or not this change in annual precipitation would change the uplift patterns in the mountains.
Borsa et al. focused on the effect of rainfall on the broader region of the American West, believing that the lack of rainfall could be affecting crustal uplift. The surface of the Earth responds elastically to loads placed on its surface: it deforms under the weight of a heavy load, and then rebounds again after the load disappears. In their study, Borsa et al. (2014) used 11 years of hydrological conditions and GPS vertical placement data from stations in the Western United States and compared the two to look for corresponding trends. Both studies excluded GPS stations in the Central Valley due to their widely variable positions in soft sediment and their presence over known and highly utilized aquifers because their motion is due to "processes unrelated to solid earth motion" (Amos et al., 2014).
The two sets of data ended up corresponding neatly, with their results showing the largest vertical displacement occurring in March of 2014 in the central Sierra Nevada, where crustal unloading of water was equivalent to 50 cm (Borsa et al., 2014). They found that uplift due to crustal unloading was most acute in regions where the lack of precipitation of the drought was felt most strongly: the coastal regions and mountainous regions that normally receive the heaviest rain and snow. The overall water deficit in the western United States was calculated to be 240 gigatons in March of 2014. Their study also explored the effects of the drought on the seismicity of the San Andreas Fault and concluded that 2 years of drought had a similar result as one week of tectonic strain, and therefore dismissed that possibility as unlikely.
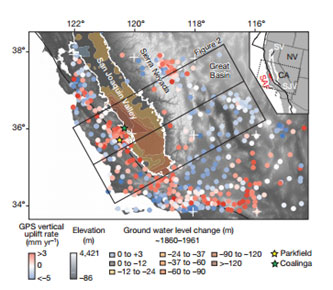
Figure 3. Contemporary GPS vertical rates and groundwater decline. Map of vertical uplift rates from GPS stations (circles) spanning California and the western Great Basin. Stations in the valley showing anomalously larger signals and local irrigation effects are excluded. Contours show historical changes in the deep, confined aquifer1. The inset depicts the tectonic configuration of the Central Valley groundwater basin (SV, Sacramento Valley; SJV, San Joaquin Valley), and the San Andreas Fault (SAF). (Amos et al., 2014)
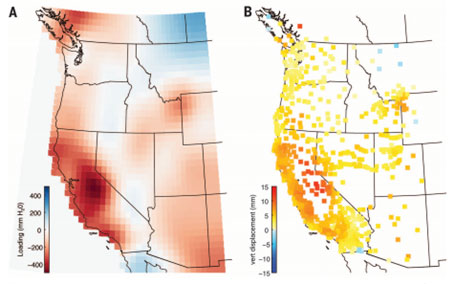
Figure 4. Maps of estimated loads and predicted displacements. (A) Loading estimate for the WUSA in March 2014. Redder areas indicate negative loading (mass deficit), bluer areas indicate positive loading (mass surplus), and white areas are unchanged. (B) Vertical displacements, corresponding to the loading model in (A), at the locations of the GPS stations used in this analysis (compare to actual displacements in rightmost panel of Fig. 1). (Borsa et al., 2014)
In their paper published in May of 2014, Amos et al. studied the effect of groundwater depletion in central California. Their claim was that the massive amount of groundwater removed from the lithosphere over the past century and a half could be having a previously unimagined effect on the seismicity of the region and crustal deformation. Roughly 160 cubic kilometers of water has been removed from the groundwater system in California in that amount of time.
Amos et al. found that vertical uplift rates were greatest along the lower western slope of the Sierra Nevada, adjacent to the Great Valley where the majority of the groundwater extraction occurs. Also, the majority of annual uplift in the Sierra occurs in the late summer and early fall, after the snowpack has had time to melt and its mass has been removed from peaks and the groundwater usage is at its highest rates down in the Central Valley due to the approach of the end of the growing season. This overall regional dryness corresponds to uplift measured at GPS stations around the edge of the Central Valley, suggesting that the isostatic equilibrium of the crust is affected (Amos et al., 2014). In the wetter spring, again these stations show a slight subsidence with the increased amount of water in the ground.
The authors of Amos et al. also studied the potential for groundwater depletion to increase regional seismicity, and their conclusion was that groundwater loss can reduce effective normal stress on the San Andreas Fault, suggesting that it may lead to a slight uptick in the frequency of earthquakes in the region (Amos et al., 2014).
Conclusion
The drought that California is currently experiencing and the removal of massive amounts of groundwater from the lithosphere are shown by multiple studies to be affecting the uplift (and potentially the seismicity) of the Sierra Nevada and the surrounding region. Even if the drought is not part of anthropogenic climate change (which many believe it is), then human beings are still having a potentially major impact on the tectonic environment in this region due to groundwater pumping. This raises many new questions about human interactions with the earth and about other ways that humans are changing the environment of which we remain unaware.
Bibliography
Amos, C.B., Audet, P., Hammond, W.C., Burgmann, R., Johanson, I.A., and Blewitt, G. (2014). Uplift and seismicity driven by groundwater depletion in central California. Nature, 509, 483–486.
Borsa, A.A., Agnew, D.C., and Cayan, D. R. (2014). Ongoing drought-induced uplift in the western United States. Science, 345(6204), 1587–1590.
California Department of Conservation. (n.d.). The California Geotour. Online Geologic Field Trip Guides. Retrieved June 15, 2015, from http://www.consrv.ca.gov/cgs/geotour/Pages/Index.aspx#sierra nevada
Davis, C.D. (2009). Uplift of the Sierra Nevada, California. Geology, 37(6), 575–576.
Department of Water Resources. (2015). California’s Most Significant Droughts: Comparing Historical and Recent Conditions. State of California. Retrieved from file:///C:/Users/Owner/Downloads/California_Signficant_Droughts_2015_small.pdf
Hill, M. (2006). Great is Granite. In Geology of the Sierra Nevada (Revised Edition, pp. 179–209). London, England: University of California Press.
Sierra Nevada Conservancy. (2011). Looking to the Source: Watersheds of the Sierra Nevada. Water Education Foundation. Retrieved from http://www.sierranevada.ca.gov/our-region/sierra-delta--connection/our-region/docs/waterreport.pdf
Sierra Nevada Conservancy. (2013, November). Drought and the Sierra Nevada. Retrieved from http://www.sierranevada.ca.gov/our-region/sierra-delta--connection/factsheets/snc_factsheet_drought.pdf
Small, E.E., and Anderson, R.S. (1995). Geomorphically driven late Cenozoic rock uplift in the Sierra Nevada, California - ProQuest. Science, 270(5234). Retrieved from http://search.proquest.com.proxyiub.uits.iu.edu/docview/213567127?pq-origsite=gscholar
State of California. (n.d.). California’s Primary Watershed – Sierra Nevada Conservancy. Retrieved June 15, 2015, from http://www.sierranevada.ca.gov/our-region/sierra-delta--connection
Tarbuck and Lutgens. (259AD). Chapter 9 Mountain Building. In Earth: An Introduction to Physical Geology. Prenhall.
Tierney, T. (2000). Geology of the Mono basin. Mono Lake Committee, Kutsavi Press.
Unruh, J.R. (1991). The uplift of the Sierra Nevada and implications for late Cenozoic epeirogeny in the western Cordillera. Geological Society of America Bulletin, 103, 1395–1404.
Zandt, G., Gilbert, H., Owens, T.J., Ducea, M., Saleeby, J., and Jones, C.H. (2004). Active foundering of a continental arc root beneath the southern Sierra Nevada in California. Nature, 431, 41–46.