Abstract
Various factors determine what can live in certain environments, but the geological processes that form the environment have a great deal of importance. In this paper, three extreme environments will be examined including alkaline and hypersaline environments, hydrothermal environments, and hot desert environments. To analyze how the extreme environments were made, information about the geological processes that created them will be included. After the geological processes of the environments are observed, the observations of the adaptations of life in those environments will be explained. Considering there are many examples of extreme environments around the world, the geological processes will be narrowed down to the alkaline and hypersaline environment of Mono Lake, the hydrothermal environment of Hot Creek, and the hot desert of Death Valley. The observations of adaptations of life in extreme environments will be in a broader sense.
Introduction
Life in extreme environments holds great importance in the future of scientific research. Research in this field will help us understand how species are evolving as they begin to adapt to harsh environments and much more. Some of the extreme environments on Earth are quite similar to environments on other planets. Remarkably, research on life in extreme environments could be useful in determining whether life on other planets is possible.
To fully understand life in extreme environments, the geological processes that create those environments have to be considered. The geological processes behind each environment are what make them unique. The variations in tectonics, structure, and volcanism in the past have resulted in diverse landforms, climate, and life in each environment.
Alkaline and Hypersaline Environments
Background
An alkaline environment is an environment with a pH above 7 while a hypersaline environment is an environment that is saltier than seawater (Bell, 2012). Organisms that live in an alkaline or hypersaline environment have developed adaptations to offset the harmful effects that are posed. Although there are environments that are solely alkaline or hypersaline, it has been discovered that some environments are both.
Observations of Geological Processes at Mono Lake: Geological History of the Mono Basin
The Mono Basin has experienced quite a bit of volcanic activity in its history and will continue to in the future. Beginning with arc-volcanism around the Mono Basin, subduction caused granite and basalt to mix from the melting sea floor plate and the melting continental plate which produced an andesitic and slightly granitic layer of rock. This mixing left an arc of volcanoes on the northwest coast. A few million years after the arc volcanoes were made; crustal stretching and faulting began which built the Basin and Range. During this time, basalt was able to make it to the surface and cover approximately 300 square miles because of the faulting and crustal thinning taking place (Tierney, 1995).
As the basalt kept rising to the surface, melted continental rock on the way up which produced a magma chamber full of granitic magma. This magma chamber was named the Long Valley magma chamber and after an immense amount of magma was built up inside, the Long Valley eruption occurred. The deposits of the Long Valley eruption, called Bishop Tuff, was spread 150 cubic miles and engulfed the southwestern Mono Basin and other areas in 600 feet of ash (Tierney, 1995).
Smaller but similar to the Long Valley magma chamber, another magma chamber was formed called the Mono Craters magma chamber. Much of the volcanism that occurs in the area today is generated from the Mono Craters magma chamber. The Mono Craters, Black Point, and volcanic islands around Mono Lake were all produced from volcanic activity from the Mono Craters magma chamber. The Mono Craters is the site of most of the current volcanism in the Mono Basin though (Tierney, 1995).
The Framework of Mono Lake
As for Mono Lake specifically, the volcanism that occurred in the past contributed to its environment that exists today. Mono Lake is a terminal lake that has no outputs. Thus water escapes via evaporation and leaves a high concentration of salt behind. The lake also contains several minerals that have seeped up from the ground. The great deals of carbonates that reside in the lake makes up its alkalinity: a pH of 10 (Mono Lake Website, 2014).
The Sierra Nevada Mountain Range acts as a rain shadow and does not allow for much precipitation to fall on the lake. Additionally, the only sources of water for Mono Lake are five fresh water streams that run into it. These freshwater streams tend to hold calcium in their freshwater. As the freshwater containing calcium mixes with the lake water high in carbonates, geological structures called tufa are formed.
Observations of the Life in Mono Lake
Overview of Life in Mono Lake
Despite high concentrations of salt and its alkalinity, Mono Lake is home to many living creatures. The most commonly known species that live in Mono Lake are brine shrimp, alkali flies, and algae. While brine shrimp and alkali flies are the most studied living creatures in Mono Lake, bacteria, protozoans, and phytobenthos also occupy its waters (Bell, 2012). Without the adaptations that these species have developed, life in an environment like Mono Lake would not be possible.
General Adaptations to High Alkalinity
To be able to survive in an environment with such high alkalinity, organisms must maintain their internal pH and the pH of their enzymes (Bell, 2012). For organisms to maintain their internal pH, also called pH homeostasis, the structure of their cell walls and cell membranes are altered. The internal pH of an organism has to stay more than two units lower than the pH of its outside environment. It has been observed that some organisms achieve pH homeostasis by sustaining a cell wall that is negatively charged. Within their negatively charged cell walls lie acidic polymers that allow the cell wall to absorb essential ions while preventing alkaline ions from entering (Bell, 2012).
The cell membrane of an organism is a second method of maintaining pH homeostasis. To do so, the cell membrane uses an electrochemical gradient which controls the amount of ions entering and leaving the cell membrane. An electrochemical gradient moves ions from a higher concentration to an area of lower concentration and vice versa. This is helpful for maintaining pH homeostasis by allowing for positively charged ions such as positively charged hydrogen, potassium, or sodium ions to flow in and out of the cell membrane (Bell, 2012).
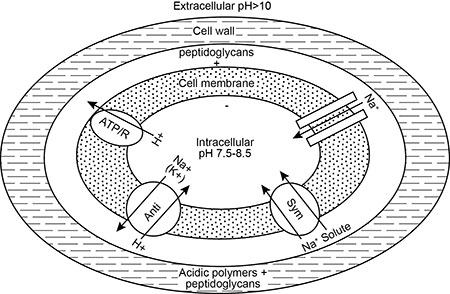
Diagram of the cell wall and cell membrane of a cell and the electrochemical gradient maintaining pH homeostasis. Figure Reproduced from Bell et al. [2012] (exact reproduction)
As organisms in environments of high alkalinity evolve, more adaptations come forth. The goal of the cell wall and cell membrane controlling the amount of ions coming in and out of the cell and the cell membrane is to keep the pH of the cell neutral. More adaptations such as enzymes evolving to become neutral and resistant to the harmful effects of different pH exist, but less is known about the evolution of the cell wall and the cell membrane of organisms (Bell, 2012).
Mono Lake is the only place in the world where these particular tufa structures are visible (Hill). These towers are composed of calcium carbonate, which is the same chemical composition that constitutes limestone (Rieger). The tufa forms underwater and has been exposed and become visible due to the lowering levels of the lake water (Young). When freshwater springs in the alkaline lake emit calcium-containing water, the calcium reacts with the accumulated carbonate in the lake to precipitate into the mineral thinolite, an unusual form of calcium carbonate (Rieger and Hill). Over time the tufa forms underwater in a column around the opening of upwelling calcium water, thus the tufa towers indicate the location of current or previous underwater springs (Rieger).
Volcanic Islands: Mono Lake has 2 volcanic islands, both of which are volcanic cinder cones (Mono Lake). The larger of the two is Paoha Island, which was formed in the mid 1600s. Paoha Island has a white surface, which is composed of lake-bottom sediments that have since been uplifted due to rising magma (Hill). The island has visible cinder cones, lava flows, hot springs, and active steam vents (Mono Lake and Hill). The smaller island is Negit Island. This island is black and was formed from a series of volcanic eruptions ranging from 1,700 to 300 years ago (Hill).
Old Lake Shores: From 1900 to 1940 the Mono Lake water level was fluctuating between 6,417 to 6,427 feet above sea level. Then Los Angeles began diverting water in 1940, causing the levels to steadily decline, eventually reaching a historic low of 6,372 in 1982 (Mono Lake Level). This exposed almost 15,000 acres of lakebed (Scoonover). These highly alkaline exposures were picked up by regular Mono Basin winds causing hazardous dust storms (Young). These exposed old shorelines are visible as concentric rings around the lake. Geologically speaking, these rings are known as strandlines. The highest strandline of Mono Basin is visible at 7,180 feet above sea level. This indicates that the lake’s water levels were once about 800 feet higher than they are today (Long Valley Caldera…).
HYDROLOGY
Inputs
Streams: 5 freshwater streams provide water to Mono Lake. The water source of the streams is the melting of the winter’s snowpack in the high Sierra Nevada. The input streams are Rush, Parker, Walker, Lee Vining, and Mill Creeks (Mono Basin Creek Profiles).
Precipitation: Another water source for Mono Lake is precipitation. The average annual precipitation at Mono Lake is a mere 35 centimeters (Mono Lake). The reason Mono Lake has such a low rainfall is due to the rain shadow effect of the Sierra Nevada Range. As the eastward moving winds rise up the Sierra Nevadas, the air expands, cools, and is less capable of retaining moisture. Therefore, along the western Sierra Nevada the majority of the moisture in the air is deposited as precipitation. After crossing the Sierra Nevada Range and continuing eastward, there remains little moisture in the air, thus accounting for the low rainfall at Mono Lake (Rain Shadow). The influence of the rain shadow effect at Mono Lake is evident as the Sierra Crest receives an average annual precipitation of 45 inches while the eastern shore of Mono Lake receives only about 5 inches (Quick Facts). Additionally, an interesting phenomenon that occurs at Mono Lake is virga. Virga is when rain falls from a cloud but evaporates before reaching the ground (Virga). The dry, hot, arid climate at Mono Lake can allow this spectacle to take place.
General Adaptations to High Salinity
For organisms to adapt to high salinity, osmosis must be regulated. Osmosis is when water is diffused from an area of high solute concentration to an area of low solute concentration to balance the concentration of solute overall (Wagner, 2014). For organisms not adapted to high salinity, water will diffuse outside of the cells into the highly concentrated salty waters and leave behind a cell that will lose its structure and function. Essentially the cell will begin to shrivel after it loses water (Wagner, 2014). To bypass problems associated with osmoregulation, organisms have developed certain adaptations like the salt-in and compatible solute strategies (Bell, 2012).
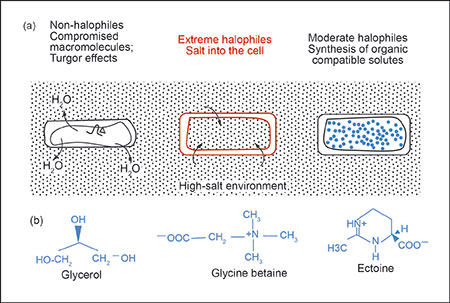
Adaptations of halophiles to hypersalinity.
Figure Reproduced from Bell et al. [2012] (exact reproduction)
Organisms that have evolved to acquire the salt-in strategy have an internal environment that has a high salt concentration (Bell, 2012). Therefore the organism is isotonic and its salt concentration is at the same level as the salt concentration of its outer environment. This prevents water from moving in and out of the cell, which regulates osmosis and maintains the structure and function of the cell in turn (Wagner, 2014). However, the issue associated with the salt-in strategy is that organisms are not able to adapt to environments with lower salt concentrations (Bell, 2012).
The compatible-solute strategy allows for organisms to adapt to an environment that fluctuates in concentrations of salt. Organisms with the compatible-solute strategy store organic compatible solutes in their cells. These organic compatible solutes regulate osmosis and do not require the organism to function in a certain manner while salt-in organisms have set ways in which they function (Bell, 2012).
Interpretation
From the observations made about the geological processes that made up Mono Lake and the observations of the life at Mono Lake, it can be inferred that there is certainly a correlation between the two. The geological processes that formed Mono Lake caused the lake to become alkaline and hypersaline. Correspondingly, the alkalinity and high concentrations of salt effected what could inhabit the area because of the geological processes that formed the environment.
Hydrothermal Environments: Hot Springs
Observations of Volcanic Processes behind Hot Springs: History of Hot Creek
Mentioned in the geological history of the Mono Basin, the Long Valley eruption had quite an effect on its surroundings. Hot creek is located in the Long Valley Caldera, which was created during the eruption. Magma under the caldera led to more volcanic eruptions and hills formed in the caldera as a result. Lava from one of the eruptions moved into a lake and while the lava was originally rhyolite, when mixed with water, it made clay and perlite. Once the lake disappeared, a stream now known as Hot Creek began to flow from the solidified magma from the eruption. Most of the water flowing into the creek originates from Sierra Nevada snow melt (Ferrar et al., 2007).
Processes behind Hot Springs
Hot Springs that reside in streams like Hot Creek are made by magma heating groundwater. Magma makes contact with groundwater through volcanic features such as calderas, fault lines, and more. Once the magma reaches groundwater, it heats the water dramatically and the water returns to the surface due to pressure (Bell, 2012). As the water moves towards the surface, it is kept from boiling because of high pressure and it loses heat from interacting with cold water. As a result of the heat loss, it moves farther away from the magma (Ferrar et al., 2007).
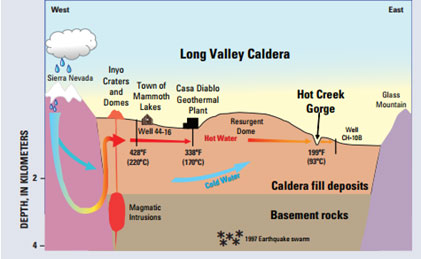
Diagram representing the hydrothermal system in the Long Valley Caldera. Figure Reproduced from Ferrar et al., [2007] (exact reproduction)
Observations of Life in Hydrothermal Environments: Overview of Life in Hot Springs
At such high temperatures, not much life is able to live in hot springs. In fact, at Hot Creek, fish moving along the stream that have come into contact with the changing temperatures of the hot springs have immediately died (Ferrar et al., 2007). It has become evident that microorganisms have evolved to adapt to the temperatures though. Microorganisms capable of living in hydrothermal environments have been named thermophiles (Bell, 2012).
Adaptations of Thermophiles
Three of the most studied adaptations to hydrothermal environments include genetic stability, protein stability, and maintaining internal pH homeostasis. Without adapting to the high temperatures, the cells of the microorganisms will denature and degrade. The organelles and other components in the cells would lose their functions as well (Bell, 2012).
To maintain genetic stability, scientists have found that the genomes of some thermophiles have been altered. Genes that code for DNA repair mechanisms have become apparent in thermophiles. In particular, they have found that many thermophiles have a gene that codes for reverse gyrase, an enzyme that assists in preventing heat and chemical denaturation (Bell, 2012).
Proteins have many functions within a cell that keep it functioning. Proteins are involved in defense, transport, cellular communication, movement, structural support, and can act as a catalyst for reactions in a cell (Wagner, 2014). Therefore, maintaining protein stability is of great importance. The structure of a protein is quite complex. Proteins are made up of polypeptides which fold and loop to make up a stable structure (Wagner, 2014). Proteins in thermophiles have shorter loops and stronger bonds to hold the polypeptides together to create a compact structure (Bell, 2012).
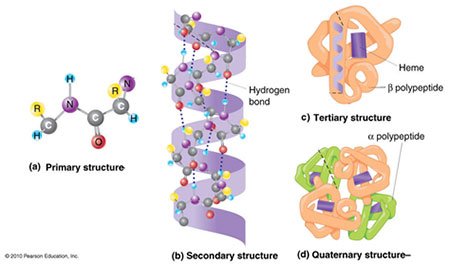
Diagram representing the structure of proteins. Figure adapted from Russell et al. [2010] (modified version)
Another modification that thermophiles have developed is the evolution of their cell membranes to promote internal homeostasis (Bell, 2012). Cell membranes allow for molecules to enter and leave a cell (Wagner, 2014). Thermophiles have developed cell membranes with a stable structure containing longer fatty acid chains that make up the membrane itself. To maintain pH homeostasis, the cell membrane of a thermophile becomes more impermeable meaning less is able to pass through. Instead there are ion transporters located on the cell membrane that essentially act as a gate to let the proper ions through to keep up pH balance (Bell, 2012).
Interpretation
The volcanic processes that create hot springs such as the ones at Hot Creek have forced adaptations among microorganisms. Without adaptations, it would be impossible for organisms to live in such a heated aquatic environment. Evidence proves that when species that have not evolved adaptive characteristics are exposed to the fluctuating temperatures of Hot Springs, they cannot survive.
Hot Desert Environments
Observations of Geological Processes in Death Valley: Geology of Death Valley
Death Valley is located within the Basin and Range Province. As stated in the history of Mono Basin, the Basin and Range was created from crustal stretching and faulting. The crustal stretching began 25 million years ago and continues to this day (National Park Service Website, 2014). Crustal stretching has caused faults to develop and for rock to move up and down them to create basins and ranges. Erosion has moved sediment from the mountains into the basins by means of water and gravity (National Park Service Website, 2014).
Relation between Climate and Geology
Considering the lowest point of Death Valley is 282 feet below sea level and it is surrounded by mountain ranges, it is difficult for heat to escape. A rainshadow prevents precipitation from reaching Death Valley which generates dry air. With dry air and little vegetation, heat is reflected off the soil and rock; it then travels back up until it is blocked by mountain ranges, and then moves back towards the surface. As the air rises it cools, but once it is recycled back to the surface, air pressure heats it up again (National Park Service Website, 2014).
On the occasion that there is rainfall, flashfloods occur and have quite an effect on the landscape. With no vegetation to prevent erosion, flashfloods can change the landscape dramatically. The rainfall is unable to be absorbed by the soil quick enough because of its abundance and leads to erosion as a consequence (Lutgens, 2005). In some instances, heavy rainfall forms ephemeral streams and playas that can last a couple of days to a few weeks. When rainfall is evaporated because of the lack of drainages, high concentrations of salt are also left behind as well (National Park Service Website, 2014).
Observations of Life in Hot Desert Environments: Adaptations to Abiotic and Biotic Factors in the Desert
The abiotic and biotic stress factors that are presented in the desert environment pose a threat to its inhabitants. Desert organisms that can successfully remain in the environment are called xerophiles (Bell, 2012). Xerophiles have adapted to the stresses of a desert environment including the dangers of radiation and temperature, the availability of water, high salinity, and wind and dust storms (Bell, 2012).
Plant Adaptations to Abiotic Stresses
There are various ways in which plants have adapted to abiotic factors in desert environments like Death Valley. Water scarcity is among one of the biggest problems for plants in deserts. To preserve and intake enough water, some desert plants have deep roots to access water that has seeped deep into the ground (Montooth, 2013). Deep roots can also help stabilize the plant during dust and wind storms.
Other plants use Crassulacean Acid Metabolism or CAM. With the CAM method, plants are able to preserve their water by closing their stomata during the day and opening them at night (Wagner, 2014). Opening their stomatas during the day could lead to evaporation of the water they are attempting to preserve making CAM plants efficient.
Radiation and temperature can also be very harmful to life in deserts. Some plants have wax or hair on their surfaces to directly reflect light (Bell, 2012). Plants have also been known to adapt by altering their photosynthetic process. Plants use methods such as photorespiration and to make use of the excess light absorbed. Photorespiration is said to wastefully use energy though, and to avoid the problems associated with photorespiration, some plants will use a strategy called the C4 photosynthetic pathway (Bell, 2012).
Animal Adaptations and Strategies against Abiotic Stresses
Animals face the same abiotic problems that plants face in desert environments, but adapt in different ways. For example, animals have adaptations like pale skin color that is associated with the lesser absorption of heat. Animals will also limit the amount of exercise done on hot days to avoid overheating. To separate the ways in which animals adapt to the desert climate, they can be split into three categories: avoiders, evaporators, and endurers (Bell, 2012)
As their name suggests, avoiders avoid radiation by using their surroundings. Avoiders will hide in areas covered in shade or underground. Lizards were thought to have some sort of genetic tolerance to heat, but new studies suggest their use of shade is the main factor protecting them against heat (Sunday et al., 2014). On the other hand, endurers are capable of withstanding the heat because of their genetic adaptations and evaporators use evaporative cooling (Bell, 2012).
Interpretation
Several abiotic factors impact the life in desert environments. All deserts have geological processes that affect the climate. The Basin and Range not only has dramatic geological history, but a dramatic climate that creates its unique environment. It is yet another example of how geology dictates biology in extreme environments.
Conclusion
Of all the extreme environments mentioned, they all have something in common. The geology that created the environments has influenced the life that lives in them. There may be extreme environments that are difficult to survive in, but life still manages to exist in them. Organisms and animals have been able to evolve to adapt to these extreme environments, and research on these marvelous creatures has been useful to the scientific community.
The ability of organisms and animals to adapt to extreme environments gives scientists hope that other organisms and animals will have the potential to evolve to changing environments in the future. Life in extreme environments is also heavily studied in hopes of determining whether there could be life on other planets. If organisms and animals can survive harsh environments on earth, there is a possibility of life on extreme environments similar to ours on different planets.
Finally, studies of life in extreme environments will aid in learning about the evolutionary history of organisms and animals. By comparing the extreme environments that are similar to the environments in the past, it is possible to get an idea of the adaptations organisms and animals had. There are endless studies that can be done on life in extreme environments, and as these studies progress, the results could be revolutionary.
List of References
Bell, Elanor. Life at Extremes: Environments, Organisms, and Strategies for Survival. Wallingford, Oxfordshire, UK: CAB International, 2012. Print.
Farrar, Christopher D., William C. Evans, Dina Y. Venezky, Shaul Hurwitz, and Lynn K. Oliver. "Boiling Water at Hot Creek–The Dangerous and Dynamic Thermal Springs in California’s Long Valley Caldera." Boiling Water at Hot Creek–The Dangerous and Dynamic Thermal Springs in California’s Long Valley Caldera. USGS, 2007. Web. 16 June 2014.
Montooth, Kristi. "Plant Adaptations to Environmental Challenges." Biology L111. Jordan Hall, Bloomington. Oct. 2013. Lecture.
"Quick Facts About Mono Lake." Quick Facts About Mono Lake. Mono Lake Committee, n.d. Web. 16 June 2014.
Russell, Peter J. IGenetics. San Francisco: Benjamin Cummings, 2002. Print.
Sunday, Jennifer M., Amanda E. Bates, Michael R. Kearney, Robert K. Colwell, Nicholas K. Dulvey, John T. Longino, and Raymond B. Huey. Thermal-safety Margins and the Necessity of Thermoregulatory Behavior across Latitude Elevation 111.15 (2014): n. pag. Proceedings Of The National Academy of Sciences of the United States of America. Web.
Tarbuck, Edward J., and Frederick K. Lutgens. Earth Science. Upper Saddle River, NJ: Prentice Hall, 1997. Print.
United States. National Park Service. "Geology." National Parks Service. U.S. Department of the Interior, 29 May 2014. Web. 16 June 2014.
Wagner, Richard. "Amino Acids and Protein Structures." Biology L112. Jordan Hall, Bloomington. Feb.-Mar. 2014. Lecture.
Wagner, Richard. "Photosynthesis: Calvin Cycle." Biology L112. Jordan Hall, Bloomington. Mar.-Apr. 2014. Lecture.