Approximately 760,000 years ago a series of eruptions in the California’s Eastern Sierra Nevadas produced an extensive ignimbrite known as Bishop Tuff. The 600 km3 area in which Bishop Tuff was extruded upon forced a 16 x 32 km (20 x 10 mi) ring fault system to collapse itself into a depression where an old magma chamber had existed (Volcanic Hazards – USGS). This area is now known as the Long Valley Caldera. Today, Long Valley Caldera is one of three calderas (Yellowstone caldera in Wyoming and Valles caldera in New Mexico) large and young enough to still have some residual magma in its chamber (Bailey, 1976). Bishop Tuff is a major component in understanding the formation of calderas, products and behavior of major explosive volcanoes, as well as the causes and effects of pre/post caldera magmatic activity within the Long Valley magma chamber. This report aims to provide an overview of the work done by several volcanologists such as Roy A. Bailey and Wes Hildreth. Their work resides in describing the extent the various deposit sections of Bishop Tuff’s chemical composition, mineralogy and temperature gradients. This information allowed volcanologists to be successful in describing ambiguities of the unique compositional zoning found within the Bishop Tuff deposits. This report also explains the fundamentals of Bishop Tuff and expands on the importance of Bishop Tuff’s siliceous composition and how the smaller, more mafic melts from the pre/post calderas did not cause a massive caldera collapse.
Geologic Setting of the Long Valley Caldera (Introduction)
In order to understand the Bishop Tuff, one needs a general background of volcanoes as well as the geological setting of the Long Valley Caldera. In terms of volcanoes there are several hazards associated. Volcanic hazards include explosions, lava flows, gas leaks, ash clouds, and several other processes that effect areas directly and indirectly. Bishop Tuff shows two main products of explosive eruptions; airfall tephra (ash falls) and pyroclastic density currents (PDCs), that are incandescent, ground hugging clouds of pumice and dust that spread out over existing topographic areas from the eruption columns which can extend hundreds of kilometers at a time (Francis, 2010). In some cases, the amount of material extruded in collaboration with short amount of time it takes for the extrusion to happen causes the magma chamber roof to cave in on itself, forming calderas as big as the magma chamber (Grotzinger, 2010). The Long Valley Caldera exemplifies these processes: (1) extrusion of ash falls and PDCs (Bishop Tuff) roughly 760,000 years ago that extend for 600 km3 across areas of California, Nevada, and as far East as Kansas and Nebraska and (2) shows evidence of an old magma chamber that existed in a ring fault system based on the massive depression (Bailey, 2004). The fault system runs along another north–south running fault system of the Sierra Nevada’s region (Bailey, 2004). And this north–south running fault system corresponds to tectonic activity on the western edge of the North American Plate as the Pacific Plate is subducting while moving northward in relation to the North American Plate (Hill, 1985). This movement has caused major deformation (stretching and thinning) to most of the Western United States, giving the classic Basin and Range topography (Hill, 1985). Of course where there is faulting, there are areas for magma transport from subducting plates to rise to the surface (Hill, 1985). This is where one can find the Long Valley Caldera.
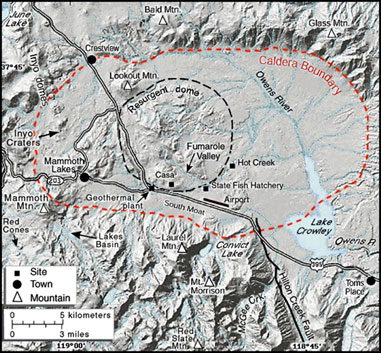
Figure 1. Reproduced from Dave Hill et al. [2008]. Image showing the area of the Long Valley caldera and surrounding landforms.
Bishop Tuff
Regional exposures of the Bishop Tuff can all be found within the areas discussed above. Roy A. Bailey postulated that most of the Bishop Tuff PDCs were carried by winds (Bailey, 1976). The most notable areas of Bishop Tuff are the Owens River Gorge which surrounds the Owens River as well as the Volcanic Table Land to the southeast of the caldera (Bailey, 1987). According to Bailey, two major components are found in these deposits: plinian ash falls (the bulk of the material) and hot incandescent ash flows (PDCs) (Bailey, 1987). This is seen extensively in a Bishop Tuff Pumice Quarry near Bishop, California. Based on the author’s field notebook, the bedding extends for about 5–6 meters high and run in and out of the bed rock (potentially product of mining). The bottom 3 meters has extensive amounts of pumice lapilli of different sizes (running 0.1 cm – 3cm in diameter) in a poorly sorted, layered, fine grained matrix indicative of plinian ash falls. Within these deposits we see areas with different clast sizes which indicate three separate eruptions varying in intensity. If one were to divide a section of the ash falls, the middle section (roughly 1 meter tall) has the most course grained rocks indicating a stronger surge. The top and bottom sections, roughly 1 meter and 1.5 meters respectively, have smaller fragments in relation to the middle, indicating less powerful eruptions. The remaining top layer is embedded with very fine sand-like grains comprised of varying clast less than 5 cm in diameter. The layer is cemented together from the hot incandescent ash flows Bailey described (Yohler, 2013). These flows are known as welded tuff, where the temperature of the flows were immensely hot causing them to fuse together (Francis, 2004).
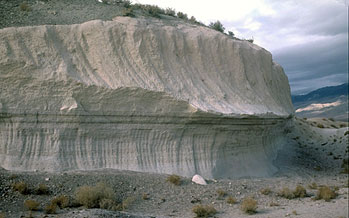
Image taken from Marai Brumm et. al [2008]
These layers only slightly describe the extent of the information gathered from Bishop Tuff deposits. In fact, Wes Hildreth analysis of the Bishop Tuff describes the nature of the Bishop Tuff in eight different eruptive "emplacement units" from the whole 600 km3 area the Bishop Tuff extends (Hildreth, 1979). (1) The first is the air-fall pumice described at the Bishop outcrop above. (2) The eastern most section is what is known as the Chidago Lobe characterized by its flows over the low north of the Casa Diablo Mountain into the Chidago Canyon and Benton and Laws. This area is also known for its lack of pyroxenes. (3) The Owens River Gorge and Rock Creek Gorge characterized by its alternating welded areas and lack of pyroxines in the lower sections that will be discussed later. These gorges are on the opposite side of the Chidago deposits. (4) The Volcanic Tableland which overlays the tops of the gorges for tens of meters. This deposit generally has two-pyroxine bearing units, but some ambiguities remain with no pyroxines at all. (5) Adobe Valley Lobe is an ash flow deposit to the north of the caldera around the western shoulder of Glass Mountain and into the Adobe Valley. This deposit contains two–pyroxenes in every sample. (6) The Mono Basin Lobe occupies the northwest section of the caldera into the Mono Basin. Hildreth describes the outcrop to be 3/4 concealed by the Quaternary deposits of Mono Lake and Mono Crater volcanoes. He also proposes this area to be deposited simultaneously with the Adobe Valley deposits with the evidence of this being discussed later. (7, 8) The San Joajuin Lobe is described by a lower cooling unit and an upper cooling unit (deposits 7 and 8 respectively). These two layers have been heavily glaciated with the 7 remaining erosional outcrops in the Middle Fork of the San Joajuin River. The layers themselves extend through a low pass in the area of Mammoth Mountain, a post–caldera structure. The lower unit is roughly 150 m thick and extends 3–12 km southwest of the caldera rim and is presumed to have gone farther before erosion. The upper unit is roughly 60 m thick. Both sections are welded together (Hildreth, 1979). The reason why pyroxenes (a mineral common in felsic rocks) were mentioned was to demonstrate varying levels of granitic composition from one layer to the next (Grotzinger, 2010). The importance of this will be discussed further on.
Temperature Analysis for Bishop Tuff Deposits
The temperature analysis by Hildreth provided several key proponents to understanding the nature of the Bishop Tuff: (1) distinguishing sub–layers in his 8 deposits described above that also agreed with Bailey’s "hot incandescent ash flows" (Bailey, 1987) and his welded tuffs from his 1976 analysis (Bailey, 1987). (2) Provide reasoning for various minerals and chemical compositions in Hildreths 8 deposits. (3) laid out eruption sequences and provided evidence for certain magmatic zoning. Generally, temperatures are a major component in a magma chamber. The Bowens Reaction Series put a temperature value when a certain mineral should begin to appear out of a melt (Grotzinger, 2010). As we will see, varying temperatures in the stratigraphic layers of Bishop Tuff yield slight mineral and chemical variations.
The temperature values were calculated by using Fe-Ti oxide in 68 samples. Hildreths calculations were based on previous work of I.S.E Carmichael in 1967 which he then compared their data (both Hildreths and Carmichaels) with interpolated curves from data of Buddington and Lindsley in 1964. What they were looking for was an equilibrium temperate and oxygen fugacity. The data showed that the phenocryst equilibrium temperature of the discharging magma increased as deeper levels were erupted. Hildreth found that the lowest temperatures were 720°C – 725°C (corresponding to basal ash fall) and the greatest temperature at 790°C at the stratigraphically highest point in a given outcrop. This agrees with the data collected, lower temperature material on bottom, higher temperature material on top. There were only 4 samples within Owens River Gorge that showed the reverse of this in between 723°C – 735°C. He concluded the range of 725°C – 790°C were also the temperatures hot enough to weld material together. When comparing these values to the layers at Hildreths and Baileys sections, we find a correlation to prove these hypotheses.
Although no temperature values were given for Bailey’s interpretation of the Bishop Tuff outcrop in Bishop mentioned earlier, one can still see the insight Hildreth provided. The bottom section of outcrop is lower-temperature ash falls while the material on top is high–temperature welded pyroclastic flows. As for Hildreth’s eight sections, values were given that also show this behavior. (1) Air–flow Pumice deposits have a cool temperature of 720–725°C. (2) Chidago Lobe was 723–737°C. (3) The two gorges: Owens River Gorge and Rock Creel Gorge shows values of 725–736°C. The values of the Chidago lobe and the two Gorges support Hildreths idea that these units were laid down simultaneously. (4) The Tableland shows an interesting behavioral side to the eruptions. With values ranging from 737°C in underlying lobes to 763°C in upper units suggest a continuous eruption which did not allow for phase re–equilibrium that will be discussed in the next section. (5) The Adobe Valley Lobe yielded the second highest values, from 760–781°C. (6) The Mono Basin Lobe yielded the highest values, from 756–790°C. The close temperature values between the Mono Basin and Adobe Valley Lobes led Hildreth to postulate a simultaneous eruption. (7, 8) The San Joaquin Lobes (the lower and upper cooling units) exhibit the lower–cooler, higher–hotter, pattern described in other outcrops. The lower cooling unit had temperatures in between 725–728°C while the upper cooling unit had a range of 772–773°C (Hildreth, 1979–all temperature values).
Brief Summary of Eruption Sequences
The beginning phases of eruptions created the initial layer >100 km3 of the low–temperature ash–fall tephra. This was then followed by the major ash–flow lobes to the southwest, east, and southeast, covering several topographically low areas. The Tableland covered the area southeast of the caldera. This is where the first phenocryst of augite and hypersthene are seen. This section was successful in destroying previous intercaldera air–fall and being welded in the most proximal areas. After the Tableland, the two north lobes (Adobe Valley and Mono Basin) were laid at simultaneous times (based on temperature range). Right before the north lobes, Hildreth believes the San Joaquin lobes were laid down. There is some discrepancy here as to whether or not this was also laid down simultaneously with the northern lobes due to very similar mineralogy and a close range in temperatures.
In terms of temperatures, this has already been briefly discussed. A continuous event in these steps: (1) explosive airborne tephra, (2) ash flows escaping to the east and southeast, (3) the discharge of hotter and deeper ash to the north. The generally movement from south-north follows the ring fault system that may have been systematically opening while other eruptions were taking place.
Bishop Tuff Chemical Analysis
Bishop Tuff presents several lines of chemical evidence that distinguishes it from pre/post caldera deposits and provide an insight as to why it caused a caldera to form. It is well known that the extent of a caldera’s eruption is due to a very viscous, gas filled, extremely siliceous magma chamber (Grotzinger, 2010). These three ingredients cause an extreme buildup of pressure, which seen by the about of ignimbrite around calderas, and force a major explosive event to occur. This sets the stage for why the Bishop Tuff is regarded as the ignimbrite of the caldera that caused its collapse. In a special report given by the Geological Society of America, Hildreth produces chemical evidence for this hypothesis. Hildreth took the mineralogy dated collected from C. M. Gilbert (1938) and M. F. Sheridan (1965) and went deeper into the compositional data in the sites listed above. Gilbert and Sheridan found common granitic minerals, quartz, sanidine, oligoclase, biotite, tanomagnetite, ilmenite, zircon, and apatite were present in every sample collected (Hildreth, 1979).
Hildreth did noticed some trends in relation to temperature changes discussed above. As progressive tapping of the magma chamber continued (the hotter the magma was) there was a drop in silica of the whole rock chemistry (>77% – >75%) (Hildreth, 1979). However, the drop in silica was not significant enough to cause a major compositional change from rhyolite to rhyodacite. On the other hand,K2O, CaO, MgO, TiO2,P2O5, and Fe increased considerably (Hildreth, 1979). This comes with no surprise seeing how Bailey noticed a mafic–felsic–mafic transition in between various stages of pre–caldera–Bishop Tuff–post–caldera of the magma chamber that will be discussed later on (Bailey, 1692).
Trace elements were also examined in which Hildreth believed showed more insight into the behavior of the magma than did major elements. For example, Light Rare Earth Elements (LREE) were enriched as the temperature increased with the opposite happening to Heavy Rare Earth Elements (HREE) (Hildreth, 1979). To put it into perspective, Ba, Sr, Eu, Mg, and LREE seemed to have been depleted from the magma chamber roof while Nb, Mo, Sb, Ta, W, U, Y, and HREE were highly enriched on the roof of the magma chamber (Hildreth, 1979). The analyzed elements were predominantly in the liquid fraction, roughly 75–95% of the magma at the time of the eruption. The following section will point out the implications of this.
In addition to chemical analysis, Hildreth noticed SiO2 gaps in several "zoned" pyroclastic flows. These gaps ranged from 2% to 10% in more silica than in other sites. There also appears to be a correlation with other trace elements as well. These indicate a long-lived interface within a magma chamber in large systems such as Long Valley. Even more interesting is that these gaps seem to follow a pattern, less-silica magma on bottom and more-silica magma on top in certain stratigraphic layers (Hildreth, 1979). These patterns in temperature, chemical concentrations, and layers in which deposited all point to magmatic zoning.
Zoning in the Long Valley Magma Chamber
Mineral composition and temperature values are important to magmatic behavior. In the preceding paragraphs the word "behavior" was used when describing temperature and chemical analysis. This "behavior" applies to Hildreths main hypothesis of his paper that the Long Valley magma chamber went through the process of zonation, or simply put, having distinctive regions, or zones, within the magma chamber. According to Volcanoes, a textbook by Peter Francis and Clive Oppenheimer, a ’standard’ model of a zoned magma chamber shows that the first erupted products are the most evolved followed by a decrease in magma evolution as the magma chamber is further tapped. This is reflected in the stratigraphic layers, where Long Valley stands out as a unique, opposition of the “standard model” (Francis, 2004). Bishop Tuff shows that the first phase of the eruption had less evolved magma from the margin of the magma chamber. More evolved magma came as the chamber was tapped from the roof, which was then laid on by less evolved magma, and then more evolved magma. As different stages of the eruption was taking place, different levels of the magma chamber were tapped, and new vents along the ring fault system opened, leaving behind a very complex variant of a zoned magma chamber (Francis, 2004). For example, the enrichment factors ("ratios of elemental concentrations in samples erupted early from the chamber’s roof zone to concentrations in samples from the final hottest level tapped") of the trace elements showed a major redistribution of minor elements that took place before the Bishop Tuff eruption (Hildreth, 1979). These correspond to the depletion of LREE and the enrichment of the HREE described above.
Also in Hildreths work, he proposes the origin of this unique zonation based on the evidence collected in the field. These conclusions led him to believe that the Bishop Tuff shows a sequence erupted from a single continuous volume of magma rather than from discrete bodies that are not in equilibrium (Hildreth, 1979). The following paragraphs are his reasonings to this belief.
- The chemistry of the phenocryst distribution show no signs that the compositional zonation should be attributed to the settling of the crystals inside the deposits. By means of fractional crystallization (Grotzinger, 2010), Zircon is the earliest crystallized silicate. However, in Bishop Tuff material, Zircon is completely unzoned and homogenous. Every grain size is the same, indicating that no separate setting velocities and re–equilibrium took place prior to eruption. This same trend is seen in many other minerals such as apatite, titanomagnetite, ilmenite, feldspar phenocryst, and various trace minerals. Pyrrhotite, orthopyroxene, and clinopyroxine appear above all sample with a temperature of 737°C but do not show settling due to their size and shape. Similarly, allanite does not appear below 763°C, but should if settling took place because of its high density. Hildreth attempted to find at least some small amount of settling by doing least-square mass-balance computations and found no combination of fractionating phases for either major or trace element gradations (Hildreth, 1979).
- Assimilation, turning mafic magma more felsic as it rises to the surface (Grotzinger, 2010), is also not the cause. Hildreth took work from Bailey and others to show that the roof of the magma chamber were mostly Mesozoic granites as well as three granitic plutons that surround the caldera. The concentration of Ba and Sr in these areas are 1, 800 and 500 ppm, respectively, while the earliest Bishop Tuff layers having only around 10 ppm. In addition there is a 20–fold difference in the amount of EU concentrations. This alone proves that the chemical differences are "inconsistent" with what assimilation should produce with feldspar rich granite.
- To complement the assimilation idea, Hildreth showed that the density and thickness of the rhyolitic basement rock would not have been penetrated by intruding basaltic magmas. If this were the case, one would expect to see large–scale crustal fusion and a unique chemical evolution (such as a reverse in Sc and Mn gradients, reduction in deep–level Bishop Tuff Ba, and in increase in V, Cr, Co, Ni, and Cu concentrations). There is no evidence of any of this contamination in any Bishop Tuff deposits. Moreover, this is not the cause of the compositional zonation (Hildreth, 1979).
- Liquid Immiscibility is also ruled out. If this had taken place, one would expect to find trace–element gradients "arising directly or indirectly" from the equilibrium of the connecting liquids. However, the Bishop Tuff shows small major-element changes and "continuity of essentially all compositional parameters (Hildreth, 1979)."
- Hildreth proposes that the compositional zoning of the magma chamber be accredited to progressive partial melting or enrichment of magmatic volatiles. He claims that the generation of progressively hotter batches of magma, incrementally growing upon each other, could cause some of the chemical gradients documented. But, due to "geologist poor understanding" of partial melting (at the time) and the anhydrous compositional gradient of the Bishop Tuff (it wouldn’t be able to stop convection at the temperature gradients given) partial melting appears to not be the cause. In addition to this, the trace–element gradients of the Bishop Tuff do not fit with partial melting theory in that the trace–elements in the partial melt and those of the solid rock would cause a large change in trace–element concentrations. The volatile component then seems more likely: water and halogens would cause the stabilization of density distribution in the melt as well as preventing any convection of the zoned part of the magma chamber. Hildreth postulates that this happened after, during, or integrated into the progressive roof ward enrichment of magmatic volatiles (Hildreth, 1979).
- Although an all–liquid explanation for the compositional zonation would be convenient, Hildreth saw this as improbable and thus proposed another cause: convention-driven thermogravitational diffusion (Hildreth, 1979). This process was coined in order to better classify large siliceous magma systems in terms of their thermal, compositional, volatile, and isotopic gradients. The data collected by Hildreth supports this, as seen by the temperature gradients, compositional features that accompany them, and the water processes discussed in issue (5) of this section.
Discussion: Why Pre/Post Caldera Magmas Didn’t Cause a Caldera Collapse (Brief Overview of Other Volcanic Activity within Long Valley Magma Chamber)
We have seen that Bishop Tuff is highly siliceous, the reason for such an explosive eruption that caused the caldera collapse. However, the magma chamber under the Long Valley area was active before and after the Bishop Tuff explosion. Bailey knew this all too well and in a special USGS report (1692), his work on pre–caldera/post–caldera features provide insight as to why the Bishop Tuff is unique within this magma chamber.
According to Bailey, the pre–caldera sequence began about 4 million years ago with widespread basalt–trachyandesite flows, ranging from the Adobe Hills in the northeast to the Central Sierra Nevadas, which evolved into more silicic dacite domes, flows and tuffs up until 0.8 million years ago (north-northwest rim of caldera around Bald Mountain and San Joaquin Ridge). There are also unique differences in the volcanic structures from west to east in which westward structures are small and scattered vents with limited flows while to eastward structures show much more voluminous lava shields and plateaus. Bailey believes this could be contributed to differences in magma source depth related to the thickness of the lithospheres beneath the Sierra Nevada and Basin and Range provinces: westward features erupting from deeper/thicker Sierran basement while eastward erupting through shallower/thinner lithosphere due to crustal thinning known in the Basin and Range Province. Generally, the mafic flows would be covered by the trachyandesite flows and include such landforms as June Mountain and Bald Mountain. In these pre–caldera episodes there is another interesting behavior: A stop in mafic extrusions leading to felsic (rhyolitic) extrusions of Glass Mountain (northeast rim of the caldera) up to 0.8 million years ago, right before the Bishop Tuff eruption. Although not discussed in Bailey’s paper, one could imagine this is possibly the first evidence of the thick Sierran bedrock truly being melted into magma.
These extrusions didn’t cause a caldera–sized eruption simply because the key ingredients weren’t there: high silica, high viscosity, and high gas. The basaltic and trachyandesite flows indicate volcanic activity that had significantly less of those materials allowing them to disperse as they erupt rather than building up and causing a rise in pressure from the gases within. In fact the pre–caldera landforms exhibit textbook examples of basaltic flows: Shields and domes (Grotzinger, 2010). As for the origin of the rhyolitic deposits of Glass Mountain, there simply was not enough material melted and extruded to cause a caldera to form. While the transition from basalt–trachyandesite–rhyolite and the exact origin of the massive magma chamber that extruded the Bishop Tuff is not well understood, Baileys and others work provide an insight to these transitions.
Post–caldera extrusions produced very similar landforms to the rhyolitic Glass Mountain, but yet again, were not large enough to produce another caldera collapse. However, there are still some unique features. These features, known as Early Rhyolite and Moat Rhyolite, formed between 700–100 ka and appear to "coeval" with other post–caldera basalt–dacite lavas erupted from vents along the moat of the caldera that interestingly enough align with the north–south trending Mono–Inyo Craters system (Bailey, 2004) .
Currently the magma chamber underneath the Long Valley Caldera continues to grow. Less than 100,000 years after the eruption, magma continued to rise and formed a resurgent dome (Volcanic Hazards-USGS). Around 600 years ago, andesite inclusions were found within the rhyolite domes of the Inyo Craters which have xenocryst consisting of plagioclase, hornblend, biotite, quartz, and sanidine that are the same composition of the phenocrysts found in the host rhyolite. From this Robert J. Varga postulated recent upbringing of basaltic magma that mixed with the porphyritic rhyolite of the Inyo domes (Varga, 1990). And post 1980 seismic activity is also attributed to activity underneath the caldera. These instances provide the insight that the magma chamber that produced the Bishop Tuff and the caldera is still active and future monitoring of this system is in order to understanding and predicting these massive explosions.
Also noteworthy is the fact that these pre/post caldera landforms do not provide the insight as to the evolution of the magma chamber as do calderas. According to Francis’ and Oppenheimer’s textbook, the only area that mentions zoning happens in dealing with "large silicic calderas" (Francis, 2004). This is largely due to the complex evolution of chemical, pressure, temperature, density, and viscosity found within calderas. It may also be attributed to the amount of material produced during a caldera: other landforms simply don’t provide a clear stratigraphic and large enough sample size to produce significant data.
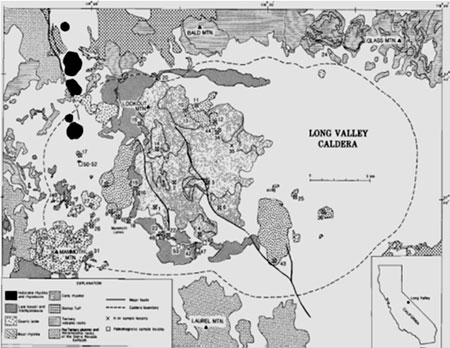
Figure 2. Reproduced from Mankinine et. Al. [1986.] Simplified geological map of the Long Valley Caldera Area with pre–caldera, Bishop Tuff, and post–caldera landforms indicated in the table.
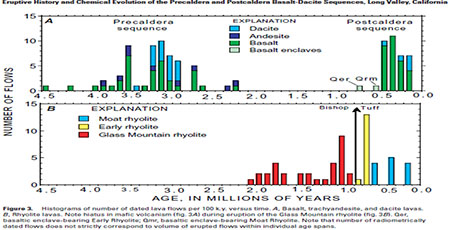
Figure reproduced from Bailey et. al. [2004]
Conclusion
What some might see as a menial, powdery substance for scrubbing one’s feet, actually provides amazing insight into volcanism. The Bishop Tuff is this example. As one can see from reading this brief overview of a few volcanist work, outcrops provide excellent clues into the behavior of a given magma chamber. Bailey and Hildreths (among many others) work in these areas showed the complex evolution of temperature and chemistry and how it effects caldera zoning processes. In addition, the bimodal magma underneath the Basin and Range provinces effect volcanism in the Long Valley area by producing several classical examples of certain volcanoes. However, this area is still active and geologists will have to continue to monitor this area and how it evolves.
Bibliography
Bailey, Roy A. "Eruptive History and Chemical Evolution of the Precaldera and Postcaldera Basalt–Dacite Sequences, Long Valley, California: Implications for Magma Sources, Current Seismic Unrest, and Future Volcanism." USGS: Professional Paper 1692. Approved 1 March, 2004. Web. 5 June, 2013
Bailey, Roy A. "Long Valley caldera, eastern California." Cordilleran Section of the Geological Society of America – Centennial Field Guide Volume 1I. Ed. M. L. Hill. Boulder, Colorado: Geological Society
of America, Inc., 1987. 163–168. Print. 10 June, 2013.
Bailey, Roy A., G. Brent Dalrymple, and Marvin A, Lanphere "Volcanism, Structure, and Geochronology of Long Valley Caldera, Mono County, California." Journal of Geophysical Research, Vol. 81, NO. 5. 10 February, 1976. Web. 5 June, 2013.
Brumm, Maria. "Friday Rock Repost: The Bishop Tuff." Science Blogs. 14 March, 2008. Web. 6 June, 2013.
Francis, Peter, and Clive Oppenheimer. Volcanoes. 2nd ed. New York: Oxford University Press, 2004. Print. 10 June, 2013
Grotziner, John, and Tom Jordan. Understanding Earth. 6th ed. New York: Clancy Marshall, W.H. Freeman and Company, 2010. Print. 10 June, 2013
Hildreth, W., The Bishop Tuff: Evidence for the origin of compositional zoning in silicic magma Chambers, Geol. Soc. Am. Spec. Paper., 180, 43–75, 1979. Print. 10 June, 2013.
Hill, David. "Bulletin of the Global Volcanism Network." Global Volcanism Program. Smithsonian National Mueseum of Natural History, October 2008. Web. 5 June, 2013.
Hill, David P., and Roy A. Bailey. "Active Tectonic and Magmatic Processes Beneath Long Valley Caldera, Eastern California. An Overview." Journal of Geophysical Research, Vol. 90, NO. B13, Pages 11, 111–11, 120. 10 November, 1985. Web. 5 June, 2013.
Mankinen, Edward A., Sherman C. Gromm, Brent G. Dalrymple, Marvin A. Lanphere, and Roy A. Bailey. "Paleomagnetism and K-Ar Ages of Volcanic Rocks From Long Valley Caldera, California." Journal of Geophysical Research, Vol. 91, NO. B1, Pages 633–652. 10 January, 1986. Web. 5 June, 2013.
Varga, Robert J., Roy A. Bailey, and Gene A. Suemnicht. "Evidence for 600 Year Old–Basalt and Magma Mixing at Inyo Craters Volcanic Chain, Long Valley Caldera, California." Journal Of Geophysical ResearchVol. 95, NO. B13, Pages 21,441–21,450. 10 December, 1990. Web. 5 June, 2013.
"Volcanic Hazards Program– Long Valley Caldera." USGS. 23 May, 2012. Web. 5 June, 2013.
V.S. McConnell, C.K. Shearer, J.C. Eichelberger, M.J. Keskinen, P.W. Layer, and J.J. Papike. "Rhyolite intrusions in the intracaldera Bishop Tuff, Long Valley Caldera, California." Journal of Volcanology and Geothermal Research 67 (1995) 41–60. Received 12 October 1992; accepted 29 September 1994. Web. 5 June, 2013.
Yohler, Ryan. "Sierra Nevada Field Notbook No. 1." 2013. Pg. 52–56. Print. 6 June, 2013.