Expansion of renewable energy technology in the Great Basin is pertinent to the maintenance of the national energy budget. Geothermal, hydroelectric, and solar power plants currently operate within the region, providing electricity to local households and industries. Power plants such as the Casa Diablo Geothermal Plant, Hoover Dam Hydroelectric Plant, and Stillwater Geothermal Solar Hybrid Plant provide insight into the state of renewable energy within the region and areas for improvement. In the coming years, regional private and public entities will likely invest in the expansion of solar, geothermal, and wind power systems in order to limit environmental degradation while maximizing local resources.
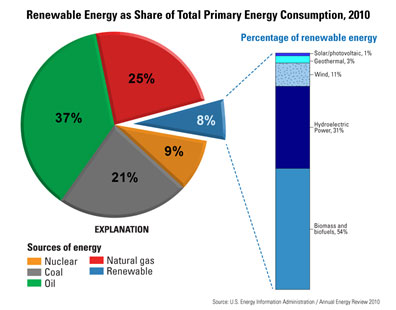
Figure 1. United States Energy Consumption Diagram. (US Energy Information Administration)
Introduction
Today, 90% of energy consumed globally is produced using a fossil fuel source (Botkin and Keller 2011). While the United States only makes up 5% of the world’s population, the nation is responsible for 40% of global oil consumption and nearly one fourth of global natural gas consumption (USDA 2008). Facing a potential end to the Oil Age due to peak oil, climate change, and environmental degradation, it is prime time for expansion of alternative energy investments, specifically renewable long–term solutions. At present, the density of power plants and transmission lines within the Great Basin region is much lower than most areas of the United States, making it a prime area for development of renewable energy technologies.
Renewable Energy Sources and Technologies
Geothermal Energy
Two forms of geothermal heat, deep–earth, high–density and shallow–earth, low–density, can be harnessed for use as electrical energy (Botkin and Keller 2011). Initially heated by the sun, shallow–earth, low–density energy is a form of solar energy that has been absorbed by Earth’s surface soils, water, and rocks. Deep–earth, high–density geothermal energy is naturally occurring heat that originates in Earth’s interior. While this type of geothermal energy is not technically nonrenewable, the energy source may be considered renewable when rates of extraction are equal to or lesser than rates of natural replenishment.
The two main ways geothermal energy is extracted by power companies include the utilization of rising steam and pumping of heated groundwater. In plants that utilize steam formed as superheated water is decompressed while rising to the Earth’s surface, steam is used to turn turbines, generating electricity (Marshark 2007). Northern California’s Geysers Geothermal Field is the World’s largest geothermal plant of this size (Botkin and Keller 2011). Plants that attain electricity directly using ground water pump that water from a production well. Once at the plant, the water transfers heat while moving through a heat exchanger, before being cooled and returned to the ground through an injection well.
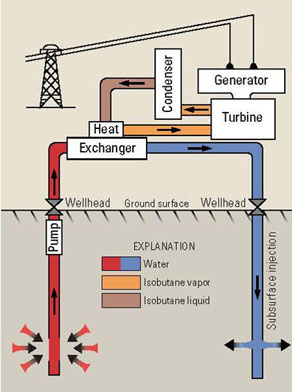
Figure 2. Simplified Diagram of a Binary Closed Loop Geothermal Plant utilizing ground water. (USGS)
Twenty–one nations currently utilize geothermal energy for electricity generation including Russia, Japan, New Zealand, Iceland, Mexico, Ethiopia, Guatemala, El Salvador, the Philippines, and the United States. Many of the nations with thriving geothermal systems are volcanically active and also feature landforms with hydrothermal alterations (Marshark 2007). For example, Iceland and New Zealand, islands notable for their regional volcanic activity, utilize geothermal energy for a major segment of their energy needs.
One risk of geothermal energy production is potential cooling of the groundwater. As mentioned earlier, geothermal energy production is only renewable if rates of heat extraction are less than or equal to rates of heat replenishment. Cooling of the groundwater not only depletes the energy source, but also can significantly impact neighboring areas. Water–cooling can damage warm aquatic environments such as spawning grounds for fish. For this reason, geothermal plants without high levels of local hydrothermal activity are likely to fail.
Solar Energy
Two types of systems exist to transform solar power into usable energy: passive and active energy systems (Botkin and Keller 2011). Active solar energy systems utilize technologies such as mechanical pumps to transport air or water, while passive solar energy systems do not. Passive solar energy systems assist in heating and cooling of buildings through manipulation of direct sunlight. For example, some individuals plant deciduous trees to shade their homes in the summer while providing direct sunlight in the winter. When most individuals think of solar energy, they depict solar collection panels and other equipment associated with active solar energy production.
Active solar energy systems utilize either solar collectors or photovoltaic cells. Photovoltaic cells, or solar cells, are composed of semiconductors and solid–state electronic components that directly convert sunlight into electricity. These installations may be used to power small operations such as meteorological stations and emergency telephones and large operations including homes and villages. Solar collectors are less direct in their conversion of solar energy into electric power. These installations work by using panels of glass that overly a black background containing a heat-absorbing liquid, such as water. The fluid circulates through tubes, transferring solar energy in the form of heat. Solar thermal generators utilize solar collectors to heat a liquid such as water in order to produce electricity. The most efficient devices use several mirrors to concentrate sunlight on a single tower containing the liquid (Botkin and Keller 2011).
Compared to other renewable energy production methods, solar energy has a low environmental impact. The main concern with active solar energy systems is the use of resources including metals, glass, and plastic in the making of solar equipment (Botkin and Keller 2011). The estimated recoverable energy provided by solar energy is approximately 75 times the amount of current global energy consumption (Botkin and Keller 2011). The vast availability of solar energy makes it a popular option for renewable energy production. The solar collector market expanded 50% between 2001 and 2004, while photovoltaics are growing at a rate of 35% per year, making it the world’s fastest growing energy source (Botkin and Keller 2011).
Wind Power
One of the most historically prominent alternative energies harnessed for production is available due to Earth’s differential heating of air masses. Wind power is employed through use of a large turbine that is forced to rotate with the wind, in the direction of the wind, mechanically generating electricity. Oftentimes, a number of wind turbines are set up along an area with abundant wind, forming a development known as a wind farm.
When utilizing wind power as a primary energy source, it is essential to place wind farms in locations with regular wind patterns, preferably steady breezes throughout the day and year. The intensity of air movement as well as the wind direction can have a major impact on the potential power attained using this technology. For this reason, wind farms are often placed either near or off of the ocean shoreline or on the top of a mountain scape where wind converges vertically in order to move over the ridge.
While wind power, like solar power, has a relatively low environmental impact, wind turbines are considered a life risk to vulnerable bird species including hawks and falcons (Botkin and Keller 2011). One benefit of wind energy is that wind farms often can allow for their land to be used for additional purposes, agricultural or otherwise. Wind energy production is increasing by more than 30% each year (Botkin and Keller 2011). While the amount of power attained using wind power systems is highly variable depending on wind speed, direction, and period, it is by far the cheapest form of alternative energy (Botkin and Keller 2011)). Globally, the estimated recoverable energy provided by wind energy is comparable to the amount utilized by humans annually (Botkin and Keller 2011).
Hydroelectric Power
Similar to wind power, hydroelectric turbines depend on the movement of a fluid, in this case water, to operate and generate electricity. Small–scale operations that produce 100kW or less are often used to power small industries, farms or homes (Botkin and Keller 2011). These systems only require downhill movement of water from a high pool to a lower pool through a generator that contains a water turbine. Larger operations also take advantage of gravity and the implication that water speed increases as a body of water moves downhill from a higher pool to a lower pool. However, larger systems regularly utilize a dam to further increase the speed and intensity of water as it moves downhill.
Dams are notorious for their damage to aquatic and terrestrial environments. In addition to obstructing the migration patterns of many fish species including salmon, they also degrade the water quality within a reservoir (Botkin and Keller 2011). By blocking the passage of fish and water, organic material builds up within a reservoir, allowing for the expansion of algal blooms and risk of eutrophication and the establishment of anoxic waters. Dams lead to the flooding of large land areas, while also increasing evaporation rates by expanding the surface area of the water. Massive flooding and drought are therefore additional risks.
Unfortunately, while the use of water to produce mechanical energy was an incredibly popular option in the 18th and 19th centuries, and has been used since the Middle Ages, modern understanding of the environmental implications of this style of power production limits its growth. Water power currently makes up 10% of energy production in the United States, but is unlikely to grow extensively in the years to come (Botkin and Keller 2011). In fact, the United States Department of Energy predicts a significant decrease in hydroelectric power generation.
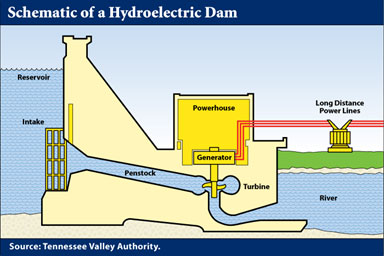
Figure 3. Diagram of a Hydroelectric Dam facility. (Tennessee Valley Authority)
Power Plant Case Studies in the Great Basin
The great basin spans many biomes and geological divides. Tensional forces between the North American and Pacific plates caused by Pacific Plate movement constructed the physiographic basin and range topography for which the American West is often revered. The Great Basin includes a great portion of this recently active province and extends west to the Wasatch Range in Utah.
In the Great Basin, hydroelectric, solar, and geothermal systems are actively used to supply energy to its inhabitants (USDA 2008). Below are three examples of regional power plants.
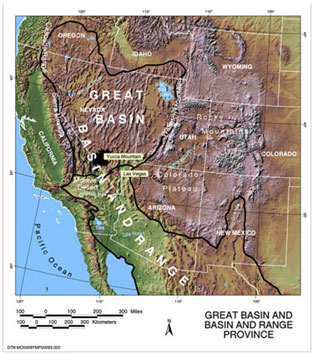
Figure 4. Map of the Great Basin and Basin and Range Province. (National Park Service)
Casa Diablo Geothermal Plant
Located near Mammoth, California, the Casa Diablo Geothermal Plant operates as a binary closed loop geothermal system. Based on the eastern front of the Sierra Nevada, the plant is located in a tectonically active region with recent volcanic activity (Hill 2006). Hot springs and fumaroles are common within the area, as well as hydrothermal alterations of rock landforms, signifying geothermal activity within the region. Because the ground water in the area is considered of moderate temperature (less than 400°F), steam is not produced at a high enough intensity to allow for a steam-dependent plant (Shallcross 2013). A production well, approximately 450 feet deep, is used to pump heated ground water (approximately 340°F) into the facility. The water then moves through six heat exchangers; heat is transferred from the ground water to isobutane through the exchangers. The isobutane, now hot and under high pressure vaporizes, turning a turbine, transferring heat energy to mechanical energy. In order to act as a closed loop the isobutane must be cooled following heat exchange in order to maintain pressure equilibrium; condensers are used for this purpose of cooling. The water that originated from the production well is returned to the ground using an injection well that is approximately 2000 feet deep.
The plant produces enough energy to power about 40,000 homes and sells its energy to a local energy utility, Southern California Edison (Shallcross 2013). The power plant, operating since 1984 under multiple management groups, represents a long–term success of geothermal power.
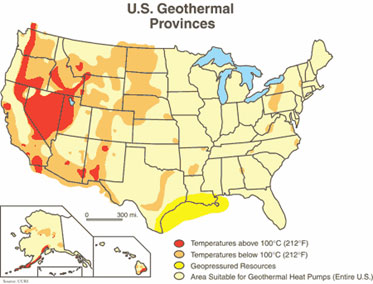
Figure 5. Map of active geothermal zones in the United States. (Texas Energy Education)
Stillwater Solar-Geothermal Hybrid Plant
Seated in Churchill County, Nevada is the Stillwater hybrid facility, a solar–geothermal facility funded by $40 million in tax support by the American Recovery and Reinvestment Act of 2009 (Neville 2012). The plant has the capacity to power about 45,000 homes in the area, providing 50 MW of energy. Managed by Enel Green Power North America, the plant has a purchase agreement with NV Energy in order to put the power generated on the grid. Originally operating as solely a geothermal plant, the previously mentioned funding through the American Recovery and Reinvestment Act of 2009 provided resources for the incorporation of solar energy production.
When operating as a hybrid, the two technologies are enhanced as daily production is stabilized, thermal efficiency is improved, and investment risk is minimized. Similar to the Casa Diablo Geothermal Plant, the site’s geothermal production operates as a moderate heat binary closed loop system (Neville 2012). Because temperatures are below 400°F, steam is not produced with enough force to drive a turbine efficiently. Therefore, the hot water pumped from a production well is used to heat isobutane, which flashes into vapor that turns a turbine, just like at the Casa Diablo Geothermal Plant. The solar portion of the plant utilizes more than 89,000 mounted polycrystalline premium photovoltaic CNPV-295 modules.
In support of the project, Steven Chu, secretary of the United States Department of Energy called the project, "the first of its kind in the world," in a statement concerning renewable energy infrastructure and technology (Neville 2012). The Stillwater Solar–Geo thermal Hybrid Plant provides insight on the importance of innovative solutions and the potential for renewable energy infrastructure development in the Great Basin. More efficient by nature, hybrid plants make energy production more economically viable.
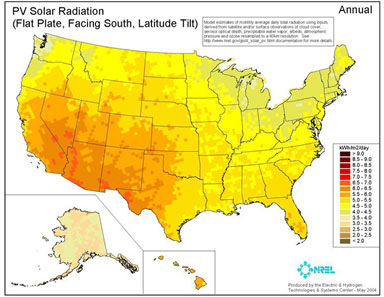
Figure 6. Photovoltaic Solar Radiation in United States. (Electric and Hydrogen Technologies and Systems Center)
Hoover Dam Hydroelectric Power Plant
Each year, the Hoover Dam, located on the Colorado River, generates 4 billion kilowatt–hours of hydroelectric power (Bureau of Reclamation 2013). One of the largest hydroelectric facilities in the world, the largest in the world from 1939 to 1949, the facility produces enough electricity to provide for 1.3 million people. Located in a U–shaped structure at the base of Hoover Dam are seventeen turbines that transfer the energy of moving water to mechanical energy, electricity.
While the dam and connected hydroelectric power facility have been remarkably successful in energy production, they have also had significant environmental impacts (Glenn et al. 1996). A study of the Colorado River Delta, performed by Edward Glenn of the Environmental Research Laboratory in Tucson, AZ and colleagues, reports severe impacts of water diversion within the lower delta of the Colorado River. As no water has been set aside for these wetlands, estuarine ecosystems in this area are in decline. Additionally, the endangerment of four fish species within the Colorado River, has led scientists such as W. L. Minckley of Northern Arizona University and colleagues to call for reform of regional water management (Minckley 2003).
Potential for Growth
Energy Production Potential
As seen in figure 5, Nevada, California, and other Great Basin states offer massive geothermal resources. Geologically active, areas within the Basin and Range province are particularly promising for geothermal energy development (USDA 2008).
As seen in figure 6, direct–beam solar radiation is readily available in the Great Basin. Regions within California, Nevada, and Utah offer the highest amounts of direct solar radiation in the nation (USDA 2008). Solar energy is a popular energy solution, and production outlook is good (USDA 2008).
While no large–scale wind power facilities currently exist in the Great Basin, the Bureau of Land Management has issued 60 wind energy testing and developing permits (USDA 2008). Energy development on public lands has become a popular option within the region.
Economic Issues
Renewable energy does come at a cost. Development of new technology and infrastructure is certainly expensive in comparison to implementing an older technology that has had decades to develop and standardize.
With that being said, Nevada spent $11 billion on energy in 2008, mostly paid to outside sources (NRDC 2003). A study by the University of Nevada Las Vegas for the state’s Renewable Energy and Energy Conservation Task Force proposes that increasing state electricity generation using renewable sources to 7% would both assist in job creation and produce $310 million in revenue annually. The study follows up that at 15%, total revenue would be more than doubled. This investment likely would have similar results in neighboring states within the Great Basin.
Environmental Challenges
In addition to the previously mentioned environmental costs associated with specific renewable energy technologies, regional production increases result in increased air, water, and noise pollution. The development of energy infrastructure also has a significant resource cost, as roads, pipelines, and transmission lines must be established. Water is another resource that is often required in massive quantities for most power plants.
Political Influence
Despite potential environmental and economic costs associated with infrastructure development, states within the Great Basin have been reported as actively encouraging renewable energy production (USDA 2008). Also, federal policies such as the Energy Policy Act of 2005 (EPACT) promote development of renewable energy infrastructure and production. The policy facilitates development through subsidies, tax credits, and research funding. While implementation of renewable energy infrastructure is controversial in some circles, political influence may allow these technologies to be more economically viable.
Conclusion
It is unlikely that the number of hydroelectric plants will increase within the region, as more economically and environmentally renewable energy sources are readily available. In tectonically active regions of the Great Basin, particularly along the Eastern Sierra Nevada range, geothermal development has proven successful, and will likely be expanded in the years to come. Additionally solar and wind power provide economically viable renewable options within the area, given consumer interest.
Key References
Botkin, Daniel B., and Edward A. Keller. Environmental Science: Earth as a Living Planet. New York, NY: Wiley, 1995. Print.
Bureau of Reclamation. "Lower Colorado Region - Hoover Dam Power FAQs." U.S. Department of the Interior: Bureau of Reclamation. N.p., n.d. Web. 11 June 2013.
Cunningham, William P., and Mary Ann. Cunningham. Principles of Environmental Science: Inquiry and Applications. Dubuque, IA: McGraw-Hill, 2006. Print.
DiPippo, Ronald. Geothermal Power Plants Principles, Applications, Case Studies and Environmental Impact, Third Edition. Burlington: Elsevier Science, 2012. Print.
Duffield, Wendell A., and J. H. Sass. Geothermal Energy: Clean Power from the Earth's Heat. Menlo Park, CA: U.S. Geological Survey, 2003. Print.
Fairley, Jerry P., and Jennifer J. Hinds. "Rapid Transport Pathways For Geothermal Fluids In An Active Great Basin Fault Zone." Geology 32.9 (2004): 825-828. Academic Search Premier. Web. 7 June 2013.
Glenn, Edward P., Christopher Lee, Richard Felger, and Scott Zenga. "Effects of Water Management on the Wetlands of the Colorado River Delta, Mexico." Conservation Biology 10.4 (1996): n. pag. Print.
Hill, Mary. Geology of the Sierra Nevada. Berkeley: University of California, 2006. Print.
Marshak, Stephen. Essentials of Geology. New York: W.W. Norton, 2007. Print.
Minckley, W. L., Paul C. Marsh, James E. Deacon, Thomas E. Dowling, Philip W. Hedrick, William J. Matthews, and Gordon Mueller. "A Conservation Plan for Native Fishes of the Lower Colorado River." BioScience 53.3 (2003): 219. Print.
Mongillo, John F. A Student Guide to Energy. Santa Barbara, CA: Greenwood, 2011. Print.
Nevada. Renewable Energy and Energy Conservation Task Force. N.p.: n.p., n.d. Legislative Report. 2003. Web. 7 June 2013.
Neville, Angela. "Stillwater Solar–Geothermal Hybrid Plant, Churchill County, Nevada." Power 156.12 (2012): 34-35. Academic Search Premier. Web. 7 June 2013.
NRDC. "Renewable Energy in Nevada." Natural Resources Defense Council. N.p., n.d. Web. 07 June 2013.
Sørensen, Bent. Renewable Energy: Physics, Engineering, Environmental Impacts, Economics and Planning. Burlington, MA: Academic, 2011. Print.
Shallcross, Jim. Personal Interview. 31 May. 2013.
USDA. Forest Service. Collaborative Management and Research in the Great Basin – Examining the Issues and Developing a Framework for Action. By Nora Devoe, Jeanne C. Chambers, and Angela Evenden. N.p.: n.p., 2008. 18 Jan. 2018. Web. 6 June 2013.