The domes and craters of the Mono–Inyo volcanic chain are a result of eruptions stemming from different magma intrusions. The North–South trend of the chain is a result of normal and strike–slip faults in the area caused by basin and range topography and the shear forces attributable to the movement of the North American plate southward relative to the Pacific plate. Various landforms developed along the chain (mainly volcanic domes, craters, and lava flows), due to differences in magma composition and the amount of gas dissolved in that magma at the time of eruption. These magmatic differences arise from different magma sources and previous eruptions of that source. Once formed, a chamber’s composition is altered by its cooling history. Because the most recent extrusions in this chain are geologically very young and are located near active hydrothermal sites, it is likely that the chain will experience further volcanic activity in the future
Satellite image of the Mono–Inyo volcanic chain. Figure reproduced from NASA [2000].
Introduction
The Mono–Inyo volcanic chain is a system of craters, domes, and lava flows that began erupting around forty thousand years ago [Alt and Hyndman, 2000], with the most recent eruption occurring about 270 years ago [Tierney, 2011]. These eruptions occurred along a line running approximately North–South. In the south, the chain begins with Red Cones and runs through Long Valley, the Inyo craters, Mono craters, and culminates at Black Point on the northwestern shore of Mono Lake. This places the chain along the western edge of the basin and range province and the northeastern flank of the Sierra Nevada Mountains. Mammoth Mountain, a large volcanic mountain consisting of a series of lava flows and domes, also lies along this chain. These flows are much older than other eruptions in the chain, as the youngest flows are about 57,000 years old [Alt and Hyndman, 2000]. The vents located south of and including Wilson Butte are known as the Inyo craters and domes, while those located to the north are designated as the Mono craters. Landforms found in this chain include cinder cones, tephra deposits, craters, volcanic domes, and coulees.
Faulting and Chain Orientation
The Mono–Inyo volcanic chain is oriented in a North–South direction, parallel to many faults in the area. Many of the landforms in the chain do not lie within Long Valley Caldera, so they are not entirely associated with ring fractures caused by the caldera collapse. Because this area is located at the edge of the westernmost basin in the basin and range province, there are many normal faults in the area running along the base of the Sierra Nevada Mountains. This is known as the Sierra Nevada frontal fault system [Riley et al., 2012]. In the basin and range, normal faults are located between the ranges and basins due to the stretching of the North American plate. As the edge of the plate is dragged northward relative to its own motion by the movement of the Pacific plate, normal faults form at the boundaries where mountain ranges are uplifted while basins move downwards. These faults provide pathways for magma to move from within the crust toward the surface.
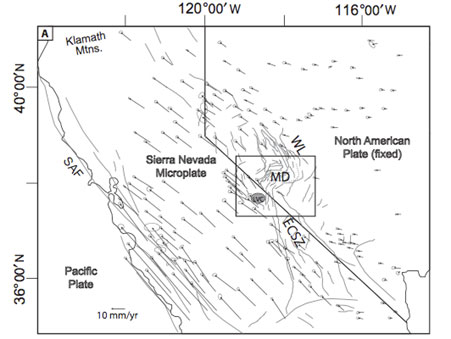
Velocities of the Sierra Nevada Microplate in the North American plate and Pacific plate shear zone. Figure adapted from Riley et al. [2012}.
Additionally, Riley, Tickoff, and Hildreth [2012] define the Sierra Nevada Microplate, which does not move along with either the North American plate or the Pacific plate. This region developed when the Gulf of California began to widen, approximately 12 million years ago [Riley et al., 2012]. The boundaries of the microplate are the San Andreas fault to the west and Walker Lane to the east, which is a depression that parallels the California–Nevada border due to discontinuous strike–slip faulting in the area. Because this microplate is within the San Andreas fault zone, it is subject to shear stress. This stress is responsible for much of the strike-slip faulting in Long Valley Caldera and the Mono Basin. These faults parallel the normal faulting of the Eastern Sierra and Mono Basin boundary, adding more fractures to the area in which the Mono and Inyo craters formed [Riley et al., 2012].
A third source of cracks that allowed magma to flow to the surface can be explained by the expansion of dikes within crustal rock. This would have taken place after magma had made its way into faults. As the resulting dike swells due to rising magma, fissures form on either side of the dike, parallel to the intrusion. If the magma volume continues to increase, more fissures are likely to form between the initial fissures. This type of fissure can be observed at "Earthquake Fault," which is a North–South running fissure between Mammoth Mountain and the Inyo Craters. With increased dike inflation, the cracked area will depress, causing the fissures to become en echelon normal faults that step toward the center of the dip. If these faults run deep enough that they contact the magma body, they provide a clear pathway for gas and magma to follow in an eruption [“Ground cracks” USGS, 2011].
Map of the location, age, and type of eruptions that occurred in the Mono- Inyo volcanic chain over the last 8,000 years. Figure reproduced from USGS, “Mono-Inyo eruptions” [2012].
Eruptions along the chain often appear in geographical and temporal groups. These groupings frequently relate to the type of magma. Some of the older eruptions, mainly those at Black Point, do not always follow the pattern of clustering but still lie along the same geographic line.
The first eruptions along the Mono–Inyo chain occurred between 300 thousand and 200 thousand years ago as basalt flows from vents near the southwestern edge of Long Valley. Around 200 thousand years ago, Mammoth Mountain began to form on the edge of the caldera from successive felsic eruptions [Bailey, 1989]. Although these eruptions piled up to create one landform, they can be seen as an eruption group because a series of flows from twelve densely located vents formed the mountain. These eruptions continued until approximately fifty thousand years ago [Bailey 1989]. The next phase of eruptive activity took place at the north end of the Mono–Inyo chain at Black Point, located in the Mono Basin. This produced mafic lava about 13,300 years ago [Tierney, 2011]. After the eruption of Black Point, activity shifted back to the southern end of the chain to produce Red Cones. This is a small group of 8,600 year old basaltic cinder cones located just south of Mammoth Mountain ["Mono–Inyo Eruptions" USGS, 2012]. The first rhyolitic Inyo dome to form was North Deadman Creek Dome, whose last eruption was about five thousand years ago [Bailey, 2004]. From approximately five thousand to four thousand years ago the Mono Craters formed, focusing mainly on those that now make up the southern Mono Craters. About two thousand years ago, Negit Island rose out of Mono Lake and shortly after, the cluster of northern Mono Craters erupted to continue the older chain of rhyolitic domes [Bailey, 1989]. Then activity shifted back to the south where the Inyo Craters erupted from 1,350 to 550 years ago. It is likely that many of these craters erupted within several years of each other [Bailey, 1989]. The most recent eruptive activity occurred at Paoha Island in Mono Lake, which was uplifted about 310 years ago and erupted around 270 years ago [Tierney, 2011].
These eruption groups suggest that craters formed near each other in time and space may have resulted from the same magmatic intrusion. Additionally, areas that experienced a lull in activity before a second phase of eruptions likely still had active magma chambers that were dormant while pressure built underground before more eruptions could take place [Bailey, 2004]. These waiting periods between eruptive groups generally last from 250 to 750 years ["Mono–Inyo Eruptions" USGS, 2012].
Volcanic Processes and Landforms
Due to the variety of explosive and effusive eruptions of different magma types along the Mono-Inyo volcanic chain, a range of different landforms is present.
Pyroclastic eruptions are often the earliest type of eruption from a young volcanic vent. This type of explosive eruption produces cinder cones and tephra deposits such as those forming Red Cones, Negit Island, and the tephra ring of Panum Crater. Also, airfall deposits of ash and lapilli have been found and are believed to have originated from the early stages of formation of the Inyo domes [Bailey, 1989]. Airfall deposits suggest much more powerful explosive eruptions than those that create cinder cones because the pyroclastic material is spread over a larger area and covers the nearby landscape. Bailey [1989] claims that the Inyo domes underwent this type of phyroclastic phase between 1368 and 1472 CE. These explosions are caused by the expansion of gas bubbles that had been dissolved in a magma as they come out of solution when the magma rises and pressure on the magma decreases. Pyroclastic eruptions can result from both mafic and felsic magmas, as demonstrated by the basalt at Red Cones and the rhyolitic nature of the Inyo domes. However, mafic magmas produce, on average, less explosive eruptions than those produced by felsic magmas, because the high silica content in felsic magma causes very high viscosity. This resistance to flow allows pressure to build underground to release a much more energetic eruption when magma reaches the surface [Alt and Hyndman, 2000]. Pyroclastic eruptions produce mainly pumiceous rocks and volcanic ash. These rocks are composed of volcanic glass (magma that cools very quickly before it can form crystals) that has been fragmented by the expansion of gas bubbles. In pumice, the gas bubbles remain trapped inside the rock while ash is blasted apart into very small pieces that become a component of airfall ["Mono–Inyo Eruptions" USGS, 2012].
Black Point also resulted from explosive eruptions, but it formed underwater when the level of Mono Lake was much higher due to glacial melt waters. Therefore, it stands as a leveled cone consisting of layers of lake sediment mixed with mafic erupted material. Most of the volcanic products in the cone are composed of palagonite, which is a brownish red rock formed by the contact of mafic lava with water [Bailey, 2004]. Later eruptions from Black Point occurred once the level of Mono Lake had dropped, because the lop layers of sediment surrounding Black Point are made up of dark grey and black volcanic ash that did not form palagointe. Additionally, the mineral thinolite can be found in the ash deposits. This mineral requires temperatures close to zero degrees Celsius to develop, so it shows that the erupted material landed in the very cold lake water after it cooled in the air during ejection [Bailey, 2004].
Common landforms found along the Mono-Inyo chain are rhyolitic domes and coulees. These occur as slow flowing rhyolitic magma extrudes from a vent that had often previously experienced pyroclastic eruptions. Initial eruptions release much of the gas that was dissolved in the magma, so the future eruptions are less explosive. As high silica lava surfaces inside of the vent’s tephra ring, it cools quickly to produce rhyolite, pumiceous rock, and obsidian. The lava on the outside of the rhyolite flow cools quickly to produce either obsidian or pumice, depending on the gas content of the lava. More magma continues to press upwards through the vent, causing the cooled shell on the outside of the flow to crack into pieces that break off, giving volcanic domes their rubbly appearance [Sharp and Glazner, 1997]. These types of domes can be found at Obsidian Dome, Wilson Butte, and other rhyolitic extrusions along the Mono–Inyo Chain. When a dome overflows its tephra ring, it will begin to flow across the surface rather than enlarging the dome. This formation is called a coulee, exemplified by Glass Creek Dome, which first erupted at the edge of Long Valley and flowed downward into the caldera. Rhyolitic coulees are also present in the Mono Domes.
An overview of the steps that form a rhyolitic dome. a) Magma rises toward the surface. b) Pyroclastic eruptions form a tephra ring. c) Pumice erupts, releasing the remaining gas from the magma. d) Obsidian forms as lava cools quickly. e) Erupted rock beings to crack as it is pushed from underneath. f) Rock continues to extrude and crack to form a dome and eventually cools, acting as a plug to contain future lava. Figure reproduced from Wholets and Heiken [1992].
Panum Crater, located near the southern shore of Mono Lake, is a young crater in which both the tephra ring and volcanic dome are visible. It is known as a polygenetic landform because it resulted from several stages of eruption. The tephra ring of Panum Crater contains smooth pieces of granite, as well as pumice, ash, and obsidian lapilli. The granitic pieces originated from the Sierra Nevada batholith and would have been deposited on the ground by Mono Lake and later, Rush Creek, after the lake level had subsided [Sharp and Glazner, 1997]. Their presence in the tephra ring shows that the initial phases of Panum Crater not only produced pyroclastic materials, but also blasted away much of the rock surrounding the vent. This would have created a crater, which was later filled in with tephra and extrusions. Next, a glassy dome began to form in the center of the crater from pumiceous obsidian crumbling downward as rhyolitic lava erupted. This is the same type of process that formed larger domes in the chain. However, a second explosive phase at Panum Crater destroyed the primary dome when gas and pressure again built up beneath the crater. The explosion breached the northeast side of the tephra ring and sent a cascade of pyroclastic material and ring tephra toward Mono Lake. Pyroclastic eruptions that followed partially filled in the newly formed crater and the break in the rim and eventually extruded a new rhyolite dome. The current dome consists of pumice and obsidian spires that were created when the crust of cooled lava fractured during eruption, so lava was forced outward through the cracks. The most recent eruption at Panum Crater occurred between 1325 and 1365 CE [Sharp and Glazner, 1997].
Craters formed due to phreatic, or steam, explosions are also present in the Mono-Inyo chain. During this type of eruption, little to no new rock is created from magma. Instead, existing rock is blasted out by hot water and other gases as they expand into steam. When groundwater contacts or nears a hot magma chamber, the water is heated and therefore rises through cracks in the overlaying rock. This water is often superheated, reaching temperatures above two hundred degrees Celsius [Rinehart and Huber, 1965]. Because this water is under pressure from the rock above, it does not boil away. When the water ascends, the pressure on it decreases and thus the boiling point lowers. Once the water reaches a certain point it vaporizes and expands rapidly, expelling the rock above it. This creates a debris mound consisting entirely of previously existing rock that was displaced with a crater in the center. The Inyo Craters are three craters formed by phreatic explosions on the flanks and near the top of Deer Mountain within Long Valley Caldera. In this case, the magma chamber either was not under enough pressure to be squeezed to the surface or it did not find its way into fractures that would lead it to erupt. The first of the three craters to erupt was the summit of Deer Mountain, the smallest crater of the three. This explosion likely caused fracturing in the nearby rock, which allowed hot water to rise and form the other two craters, located South of Deer Mountain. It is likely that the Inyo Craters all formed within several days. Using dendrochronology, these craters were found to be between eight hundred and five hundred years old [Rinehart and Huber, 1965].
This is a cross section of a small, shallow magma chamber. When magma rises into a dike or sill, the magma body will swell to form a small magma chamber. If it is close enough to the surface, pressure from the magma will push the overlying rock up so that the ground level is uplifted above the magma. Figure adapted from Wholetz and Heiken [1992].
Another type of volcanic process that has affected the chain does not always result in the eruption of magma or rapid changes in topography, but results from the rising of magma toward the surface. Swelling of the magma a chamber causes deformation of the surface and uplift over time. This is the case on Paoha Island in Mono Lake, which rose above the water level due to volcanic activity, but is mainly composed of sediments from the bottom of the lake. The island is 310 years old; the youngest volcanic eruption in the Mono–Inyo chain is located on the northern shore of Paoha Island. This dome was extruded 270 years ago, soon after the rest of Paoha Island was uplifted [Tierney, 2011]. Though the island must have begun to rise earlier than 310 years ago (so that it reached above the water level at that time), it is likely that the uplift was caused by the same magma source that created the volcanic dome. Because this process involves increasing the surface area of the ground level rock, fissures often develop that may allow magma to flow to the surface. Active hot springs on Paoha Island are possible due to this type of fracturing [Tierney, 2011].
Magma Composition and Rock Types
The wide variety of igneous rock types present along the Mono-Inyo chain results from different magma sources and different times of eruption. These magma bodies are separate from the large chamber beneath Long Valley and are of lesser age. Though some of the small intrusions may have originated as part of the Long Valley chamber, their composition is partially reliant on the depth at which they separated and the country rock through which they have traveled [Bailey, 1989]. The cooling history of these chambers also affects the composition and texture of the rocks they produce.
The earliest eruptions along the Mono–Inyo chain consisted of basaltic and andesitic flows near the southwestern edge of Long Valley, near what is now Mammoth Mountain. These flows produced landforms such as Devil’s Postpile and began to fill in areas of the caldera [Bailey, 1989]. Basalt flows in and around Long Valley are thought to have originated from basalt intrusions deep within the Long Valley magma chamber. Because basalt has a lower silica content than more felsic magmas, it has a lower viscosity and therefore travels more easily through cracks to the surface, even though it was injected below much of the felsic magma. This basalt would have created dikes, eventually making its way to vents where it was able to flow onto the surface. Red Cones is the site of other mafic eruptions near the south end of the chain. Because there is a gap of several thousand years between the initial basaltic flows and the explosive eruptions at Red Cones, they probably do not share a magmatic source near the surface even though there have been few other mafic eruptions along the chain. Also, Red Cones were created by explosive eruptions, which means that there had to be gas dissolved in the source magma. Basalt flows are effusive eruptions and therefore have a very low gas content. This further proves that these eruptions came from different sources. However, the dikes feeding them are likely derived from the basaltic intrusions in and around the Long Valley magma chamber [Bailey, 2004].
Other basaltic eruptions took place at Black Point at the north end of the chain. Because there is a geographical as well as a time gap between the basaltic eruptions in the south and those that created Black Point, these eruptions were fed by a different intrusion. It is not entirely clear where the Black Point magma originated. This extrusion predates the eruptions of the Mono and Inyo craters and is clearly comprised of basalt altered by water. Because there are no other mafic landforms near Mono Lake, Black Point’s source may have been small in volume during the eruption time [Bailey, 2004]. However, the eruptions that created Negit and Paoha islands are more mafic than most of the Mono–Inyo craters. The two islands, due to their similar composition, time of eruptions, and close proximity to each other, are thought to feed off of the same magma chamber. These cinder cones and flows are made up of dacite, which has a lower silica content than rhyolite [Bailey, 2004]. Though dacite still has a much higher silica content than basalt, it is possible that the magma chamber that created Black Point evolved over time as much of the mafic material crystallized. Because there is a time gap of approximately twelve thousand years between the eruptions at Black Point and those that began to create Negit Island, there would have been time for the magma composition to change. This also could have been enough time for the basaltic magma to cool entirely, meaning that Negit and Paoha Islands were created from a different intrusion than that of Black Point [Bailey, 2004].
The rest of the lavas along the Mono–Inyo chain have a much higher silica content than the basaltic eruptions. The earliest felsic eruptions occurred at Mammoth Mountain after basaltic flows in that area and before the eruptions at Black Point or Red Cones. The mountain is composed of overlapping quartz latite domes. Quartz latite is a fine-grained rock with a high silica content and contains quartz phenocrysts. Due to the very similar compositions of the domes, they appear to be vents that fed from the same magma chamber [Bailey, 1989].
The Mono craters are also made up of intersecting volcanic domes, but form a north to south running range rather than one large mountain. Surveys have found that these craters stem from a shared sublinear magma chamber running parallel to the craters between ten and twenty kilometers beneath the surface [Bailey, 1989]. The earliest eruptions in this area began to form the Mono craters about forty thousand years ago, forming rhyolite domes and coulees. The resulting rocks are porphyritic, containing a modest number of phenocrysts. Domes that erupted more recently (within the last three thousand years), lack the phenocrysts found in the earlier eruptions, but retain a similar overall rhyolitic composition. This suggests that the younger craters erupted at a higher temperature so that parts of the magma were not able to cool and crystallize before reaching the surface. This could also be explained by the magma originating closer to the surface than it had in previous eruptions, so that its ascent took a shorter amount of time [Bailey, 1989].
After the Mono crater eruptions, activity was concentrated at the Inyo domes. The two oldest Inyo domes, North Deadman Creek Dome and Wilson Butte, were created during the same period as some of the younger Mono craters. The rhyolites that make up these domes are alike in composition to those found in the Mono Basin. Because they are near the Mono craters chronologically, these two domes could have resulted from dikes stemming from the rhyolitic magma chamber beneath the Mono craters [Bailey, 1989].
More recently (within the last 650 years), the Inyo Craters and three more rhyolitic domes erupted near North Deadman Creek Dome and Wilson Butte [Bailey, 1989]. These newer domes are South Deadman Creek Dome, Glass Creek Dome, and Obsidian Dome. These eruptions happened in quick succession, probably in several years or less. As is usual with rhyolitic domes, the initial eruptions were pyroclastic, but these explosive eruptions terminated before any of the effusive eruptions began. There are two distinct types of rhyolite that make up the most recently extruded Inyo domes. One is light in color and density, containing many gas bubbles and large phenocrysts, similar to the Long Valley rhyolites. The other is a high silica obsidian with few phenocrysts like the rhyolites comprising the younger Mono craters. The different rock types are fused together in such a way that the rocks appear marbled, suggesting that the magmas mixed beneath the surface before they were extruded. Bailey [1989] explains that this is a result of the merging of magmas from the chambers beneath Long Valley and the Mono craters. Therefore, there must be cracks underground connecting both chambers to the magma that supplies the Inyo domes. Due to its location, it is likely that this dike heated the groundwater that caused the explosions at the Inyo Craters ["Geologic history," 2012].
Eruptions at Obsidian Dome, Glass Creek Dome, South Deadman Creek Dome, and the Inyo Craters resulted from dikes feeding off of the same magma chamber. Figure reproduced from USGS "Geologic history" [2012].
Current Activity and Potential for Future Mono–Inyo Eruptions
Although there have been no eruptions of lava in recent history, there are is evidence of active volcanism in the Mono-Inyo chain. On Paoha Island in Mono Lake, there are geothermal hot springs and steam vents that are heated by a magmatic intrusion lying below. In 1890, the lake experienced unusually large waves as well as scorching waters and gas emissions from vents on Paoha Island. These are all evidence of magma near the surface and may have been caused by an eruption within the lake [Tierney, 2011]. During the past five thousand years, eruptions have occurred along the Mono-Inyo chain between every 250 and 700 years [Bailey, 2004]. Because eruptions occurred around Paoha Island approximately 270 years ago, it is possible that eruptions will occur near there again [Tierney, 2011].
There are also active fumaroles on the flanks of Mammoth Mountain. In 2006, three members of Mammoth Mountain ski patrol were killed when they fell into a vent and were unable to climb out. This accident occurred at the largest fumarole on the mountain, which releases carbon dioxide and water vapor. Because carbon dioxide is more dense than air, it pools in depressions, such as those created around the fumarole. This causes the area around such vents to be dangerous because there may not be enough oxygen in the air [Covarrubias and Smith, 2006]. Fumaroles are evidence of magma beneath the surface, which heats and releases gasses, as well as fractures that reach deep into the earth [Bailey, 2004].
In 1989, scientists discovered trees dying on the lower southeastern slopes of Mammoth Mountain [Sorey et al., 1998]. It was later discovered that these trees were killed due to high levels of carbon dioxide in the soil, which had been released by a nearby fumarole on the mountain. It is likely that future eruptions from Mammoth Mountain would occur as mafic flows from this area or from other vents near the mountain’s base, because these are sites of past lava flows [Bailey, 2004]. Before magmatic eruptions, there is often increased seismic activity or changes in gas emissions that can act as warning signs to prompt studies like those that discovered an intrusion of magma moving within Mammoth Mountain at the time of the tree kills [Sorey et al., 1998]. Due to active fumaroles and geothermal vents within the Mono–Inyo volcanic chain, this area has a high chance of future volcanic eruptions.
Works Cited
Alt, David and Donald W. Hyndman. Roadside Geology of Northern and Central California. Missoula: Mountain Pres Publishing Company, 2000.
Bailey, Roy A. "Eruptive History and Chemical Evolution of the Precaldera and Postcaldera Basalt-Dacite Sequences, Long Valley, California: Implications for Magma Sources, Current Seismic Unrest, and Future Volcanism." U.S. Geological Survey. Reston, Virginia: 2004
Bailey, Roy A. "Geologic Map of Long Valley Caldera, Mono Inyo Craters Volcanic Chain, And Vicinity, Eastern California." U. S. Geological Survery. 1989
Covarrubias, Amanda, and Doug Smith. "3 Die in Mammoth Ski Patrol Accident." Los Angeles Times. 2006. website
"Geologic history of the Inyo Craters volcanic chain." U. S. Geological Survery. Volcano Hazards Program. 2012. website
"Ground cracks and faults associated with the Inyo eruptions." U.S. Geological Survey. Volcano Hazards Program. 2011. website
"Mono–Inyo eruptions over the past 8,000 years." U. S. Geological Survery. Volcano Hazards Program. 2012. website
Riley, P., B. Tickoff and W. Hildreth. "Transtensional deformation and structural control of contiguous but independent magmatic systems: Mono-Inyo Craters, Mammoth Mountain, and Long Valley Caldera, California." The Geological Society of America. 2012.
Rinehart, C. Dean and N. King Huber. "The Inyo Crater Lakes – A Blast in the Past." Mineral Information Service, State of California Division of Mines and Geology. September 1965.
Sharp, Robert P. and Allen F. Glazner. Geology Underfoot in Death Valley and Owens Valley. Mountain Press Publishing Company: Missoula, 1997.
Sorey, M. L., W. C. Evans, B. M. Kennedy, C. D. Farrar, L. J. Hainsworth, and B. Hausback. "Carbon dioxide and helium emissions from a reservoir of magmatic gas beneath Mammoth Mountain, California." Journal of Geophysical Research. 1998.
Tierney, Tim. Geology of the Mono Basin. Mono Lake Committee Field Guide Series. Lee Vining: Kutsavi Press, 2011.
Images
"Geologic history of the Inyo Craters volcanic chain." U. S. Geological Survery. Volcano Hazards Program. 2012. website
"Mono–Inyo Craters satellite image." National Aeronautics and Space Administration. 2000. website
"Mono–Inyo eruptions over the past 8,000 years." U. S. Geological Survery. Volcano Hazards Program. 2012. website
Riley, P., B. Tickoff and W. Hildreth. "Transtensional deformation and structural control of contiguous but independent magmatic systems: Mono-Inyo Craters, Mammoth Mountain, and Long Valley Caldera, California." The Geological Society of America. 2012.
Wohletz, Kenneth, and Grant Heiken. Volcanology and Geothermal Energy. Berkeley: University of California Press, 1992. website