Volcanic activities, especially volcanic eruptions, are presumably the most violent and terrifying natural disaster that significantly threatens human lives, properties, and natural ecosystems. Recognizing the remarkable danger of volcanic activities, this paper was prepared with the purpose of providing an overview of several volcanic hazards and the magnitude of their threats. The paper divided the volcanic hazards into two general categories based on the nature of their formations. The primary hazards are the hazards that are formed directly by eruptions or other volcanic activities and the secondary hazards are the catastrophic events that are indirectly associated with volcanic activities. In each of the two sections, the paper went through the theoretical concept of hazard formations and their possible threats. Several cases based on historical volcanic activities were also provided to present some examples on how the theoretical concepts take place in actual settings. In the final section, the paper concluded that indirect hazards should seriously be taken into consideration when analyzing the impact of volcanism since they are more deadly and widespread than the direct hazards. The paper also suggested that geologists should put more focus in forecasting catastrophic aftermaths rather than forecasting eruptions to better mitigate volcanic hazards.
Hazards Associated with Volcanic Eruptions
Despite being one of the most destructive forces in earth, volcanic eruption itself is not as threatening to human life as compared to other natural disasters like tsunamis and earthquakes. In fact, most of fatalities associated with volcanic eruptions are caused by indirect hazards rather than the eruption itself. A recent study made on the victims of volcanic eruptions since 1783 AD showed that up to 64 per cent of volcanic–related fatalities are caused by indirect hazards such as mudflows or lahars, volcanic tsunamis, post–eruption famine and epidemic disease. In comparison, only 27 per cent of death is caused by pyroclastic flows, which are a direct hazard of a volcano (Marti and Ernst, 2005)
Volcanic hazards can be divided into primary and secondary hazards. Primary hazards are direct hazards from eruption such as pyroclastic flows, pyroclastic falls, lava flows and poisonous gas release. The secondary hazards are the indirect consequences such as lahars, tsunamis and epidemic disease and post–eruption famine.
Primary Hazards
Tephra Falls
During eruptions, volcanoes release a huge amount of pyroclastic rocks or tephra into the atmosphere and these clastic deposits will fall back and accumulate on the surrounding Earth’s surface. Tephra fragments are classified based on their size ranging from ash, particles smaller than 2mm in diameter; to lapilli, fragments between 2–64mm in diameter; to blocks and bombs, rocks that are larger than 64mm in diameter (Fisher, 1961). The distribution of ejected tephra typically involves the larger and more dense blocks and bombs, which fall down the fastest and closest to the vent, while finer ash may stay in the atmosphere for days or weeks and travel thousands of miles away from the vent (Figure 1).
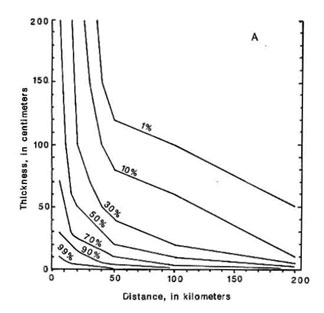
Graph 1: Graph of percentage of Tephra deposits against distance from vent (Scott, 1989)
Since tephra has the ability to travel at a great distance, even circumglobal for some large eruptions, the airfalls are the widest range of all direct volcanic hazards. Tephra airfalls pose a number of hazards. The first is large falling fragments. Small falling rocks and bombs from eruptions are usually harmless and minimal shelter is typically enough to protect victims from the falling fragments. However, large falling bombs can give substantial force of impact and may harm victims even if they are protected in a secured shelter. Falling bombs usually affect victims in a close proximity to the vent, but in some cases, the bombs could be thrown up at a considerable distance. The Mount Asama 1938 eruption, for example, ejected 1–meter diameter bombs as far as 4.5 km (Minakami, 1942). The other potential hazard is burial due to accumulation of tephra. Burial could cause building collapse, destruction in power and comunication lines and damage in vegitation. The effect of tephra burial could become worse if tephra accumulation is immediately followed by rain or snow fall because moisture by the precipitations will greatly increases the density of originally porous and uncompacted tephra. Tephra airfalls can also result in suspension of fine–grained particles in the atmosphere. These suspended particles may cause serious health problems by worsening the air quality and visibility of the affected areas. Public facilities and industries may also be affected since tephra could damage unprotected electric generators, water treatment plants and sewage system (Scott, 1989).
Lava Flows
Lava flows are the movements of molten rocks that are released from volcanic vents either through effusive or explosive eruptions. Advancing lava flows destroy everything that is on their way through razing them down, surrounding them, engulfing them over or igniting fire on them. Despite the great destructive force, death associated with lava flows is extremely rare because in most cases, lava flows move very slowly that they give enough time for victims to evacuate from the affected area (Figure 2). However, in exceptional occasions, lava flows could move much more quickly as in the case of Mt Nyiragongo, Zaire in 1977, where hundreds of people were killed by thin lava flows racing down from the lava lake on the mountain at rates faster than 60 miles per hour (Finkel, 2012). The speed of lava varies depending on several factors such as type and viscosity of lava, steepness of the slope it is flowing, flowing pattern and rate of lava production. The area affected by lava flows is also limited since they usually do not move very far from its source (USGS, 2010).
The primary hazard of lava flows is total destruction of property on the properties. Unlike other natural disasters that destroy buildings, homes, and crops, but allow the owners to rebuild them back in the same location, lava flows blanket those structures completely with tens of meters of hardened lava and replace any property lines and landmarks with new black hummocky landscape. The flows usually left their victim unable to use, sell or even locate their land. Even if the owners were able to sell the land, they typically received just a small fraction from what the land was worth before the eruption (USGS, 2010). Lava flows can also cut off the evacuation routes when they surround the victims or block roads and highways that serve as the only way out. However, since the movement of lava flows is greatly influenced by gravity, their paths can usually be projected in advance by analyzing the location of the volcanic vents and the surrounding topography (Scott, 1989). When lava flows occur underneath or within an area with glacier, they could melt snow and ice that can lead to far–reaching mudflows or lahars. This hazard will be explained in a later section.
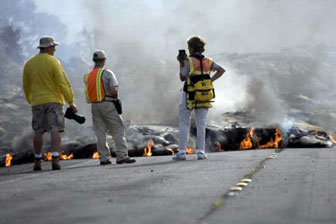
Figure 2. Two Hawaii Volcanoes National Park rangers and a local photographer watch the slow advancement of lava in Kalapana, Hawaii
Pyroclastic Flows
Pyroclastic flows are rapid streams of high–density mixtures of hot, dry pyroclastics, ashes, and gases that flow down from volcanic vents following explosive eruptions. Pyroclastic flows can also occur from effusive eruptions when parts of a volcanic dome collapse and break apart. Similar to lava flows, pyroclastic flows are deadly and destroy almost everything that lies in their path and turn the surrounding landscape into a barren land. However, pyroclastic flows move much more rapidly compared to lava flows with speeds ranging from 100 to 150 miles per hour. With that being said, the flow not only can burn anything in its path with its extreme temperature but also crush them down with its forceful momentum. Unlike lava flows, pyroclastic flows give no time for its victims to evacuate and usually leave nothing as remnant and no one as survivor (USGS, 2010).
Pyroclastic flows present a number of hazards such as destruction by rapid turbulent flow, impact by rock fragments, and burial. Additionally, hot pyroclastic flows may also cause incineration, poisonous gas, and asphyxiation. Due to their speed and amount of heat, pyroclastic flows have led to several disastrous volcanic events such as 79 AD Vesuvius eruption that killed more than 2,000 lives; 1902 Mt Pele eruption with 30,000 deaths; 1951 Mt Lamington eruption with 3,000 deaths; and 1982 El Chichon with 2,000 deaths (Scott, 1989).
Volcanic Gases
Volcanic gases are gases dissolved in magma that are released to the atmosphere during eruptions. Aside from eruptions, gases may also escape continuously to the atmosphere while magma is still underneath the ground or lies close to the surface. In such cases, gases are released through volcanic vents, fumaroles or hydrothermal systems. Volcanic gases are composed of several different types of gas and the composition varies considerably from one volcano to the other. However, water vapor consistently remains the most abundant gas released in most eruptions. Other common components of released gases include carbon dioxide, sulfur dioxide, hydrogen sulfide, hydrogen, carbon monoxide, hydrogen chloride, hydrogen fluoride, and helium. Out of these volcanic gases, carbon dioxide, sulphur dioxide and hydrogen fluoride cause the greatest hazard to humans, animals, plants and property (USGS, 2010).
Sulphur Dioxide Hazard: Sulphur dioxide is a colorless toxic gas that has a pungent and irritating odor. This gas is hazardous to human health in that it irritates the eyes, nose and throat even when small amount of the gas come in contact with the skin or mucous membranes. Inhaling sulphur dioxide is also harmful for the respiratory tract and bronchi. Over–exposure to sulphur dioxide to may lead to severe respiratory problems, difficulty in breathing, preterm birth and, in some cases, premature death (United States Environmental Protection Agency, 2013). Sulphur dioxide can also mix with water vapor in the atmosphere to form acid rain that is very corrosive to structures and landscape.
Carbon Dioxide Hazard: Carbon dioxide is another colorless gas that is harmful to lives in large amounts. Different from sulphur dioxide, carbon dioxide is odorless and can easily be diluted to low concentrations following an episodic eruption or continuous release from vents or fumaroles. Carbon dioxide poses a great hazard to human, animal and plant when the released gas is not properly diluted, leaving the surrounding area with a concentration level that is lethal to living beings. Additionally, since carbon dioxide is denser than air, the concentrated carbon dioxide subsides to low–lying areas and extremely affects the low–elevation areas (USGS, 2010). A classic example of carbon dioxide hazard is in the Horseshoe Lake Tree Kill area in Long Valley caldera, where a large amount of vegetation was killed between 1988 and 1989 due to abundance of carbon dioxide released and concentrated within the area (Figure 3).
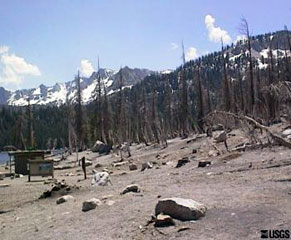
Figure 3. Horseshoe Lake tree kill area (USGS, 2010)
Secondary
Lahars
Lahar is an Indonesian word for a mixture of water and pyroclastic materials flowing down the volcanoes or river valleys. The pyroclastic materials that make up the lahar consist of unsorted volcanic debris of different sizes ranging from fine clay to large boulders. The speed of lahars varies according to their size. According to US Geological Survey, "small lahars less than a few meters wide and several centimeters deep may flow a few meters per second [and] large lahars hundreds of meters wide and tens of meters deep can flow several tens of meters per second" (USGS, 2010). These flows of mud, rocks and water seem to be like a fast–moving, wet concrete especially when they carry a large amount of volcanic debris (Figure 4). Lahars can form through multiple mechanisms, which include ice or snow melting, post–eruption rainfalls, lake failure and landslides.
The speed and size of lahars change as they go farther from their source, thus changing the type of hazards they pose. At the beginning of the flows, lahars are usually forceful enough that they could rip and drag houses, big boulders and trees for several miles. As they move farther downstream, lahars often lose their intensity and pose a potential threat of burial to the surrounding. In some cases, however, lahars could increase their intensity as they travel further from their source. The initial lahars can incorporate more water into the flows through overrunning a river or stream and melting snow or ice. Lahars can also increase the amount of debris they carry by eroding rocks and tearing out vegetation in the surrounding landscape. One of the most notorious lahar flows occurred in 1985 during Nevado del Ruiz eruption in the Andes Mountains of western Columbia. The eruption became catastrophic as hot gas released by the eruption melted the glaciers and snow on nearby mountains and produced a huge amount of water. The water combined with volcanic debris and ash to form a 40–meter deep lahar that rushed downstream at a speed of 50 kilometer per hour. The lahar flows killed more than 22,000 lives and entombed the town of Armero, which is 50 kilometer away, with 8 meters of mud (Fleury, 2009).
Tsunamis
Tsunamis are huge sea waves formed when a large volume of water is suddenly displaced above the sea level by vertical movement of the continental shelf. As the uplift in the seabed builds up the waves, the waves gain huge energy and height, and travel at a very high speed to reach the shore and crush anything at the coastal areas. Tsunamis can reach a speed of 650 miles per hour in deep water and in shallow water, the speed decreases but is still as high as 200 miles per hour. Tsunamis can be caused by various mechanisms, but volcanic tsunamis are the result of underwater explosions, lahars, pyroclastic flows, collapse or tectonic movement associated with volcanic activities (Scott, 1989). Statistically, about 5 percent of tsunamis are associated with volcanic activity and 16.9 percent of volcanic fatalities are caused by tsunamis (Marti & Ernst, 2005).
Tsunamis have been one of the most deadly volcanic hazards. Due to their massive size, tremendous speed and extensive reach, these catastrophes poses enormous destructive power on properties and result in many fatalities even at locations far from the eruptions. For example, the Krakatau eruption in 1883 triggered one of the most catastrophic volcanic tsunamis in our recorded history (Figure 5). Within a minute after the eruption, 35 m waves charged the surrounding coastline and partly destroyed almost 300 villages in the neighboring islands. The tsunamis also drowned more than 30,000 people and countless livestock to death (Marti & Ernst, 2005). The primary hazard of tsunami is inundation and the only reliable mitigation for the hazard is a tsunami warning system (TWS) that facilitates evacuation process prior to the event. However, tsunamis often build up and travel very quickly so they allow very little time for victims in to evacuate the coastal area. The evacuation process is even harder for people who live in low–lying coasts, as they have to move farther to get safety (Scott, 1989).
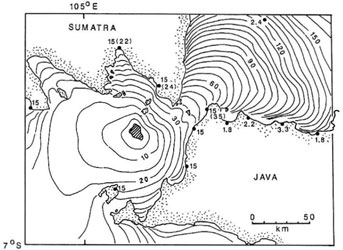
Figure 5. Map showing the travel time (in minutes) of the Tsunami generated by Krakatau 1883 eruption.
Conclusion
Based on the analysis above, there are at least three observations that we came up with. First, the hazards of volcanic eruption are not only restricted to areas in close proximity since it is apparent that secondary hazards of a volcano could travel at a great distance to reach an extensive area. For that reason, the study of volcanic hazard mitigation should not only be exclusive to countries with active volcanism, but other countries should also be involved in devising mitigation plans in order to prepare themselves for the possibility of secondary volcanic hazards. The second observation is that indirect hazards often result in a greater number of fatalities compared to direct hazards. With that being said, authorities should put the same, if not more, effort in devising mitigation plans for secondary hazards of volcanic eruptions. Finally, the number of fatalities does not correspond with the magnitude of eruption, rather, it corresponds to how catastrophic the aftermaths of the eruption are. On that account, the most important role for scientists and geologists is not to forecast enormous eruptions but to forecast catastrophic aftermaths following an eruption.
Works Cited
Finkel, M. (2012, April). Nyiragongo Volcano. Retrieved June 13, 2013, from National Geographic: website
Fisher, R. V. (1961). Proposed Classification of Volcaniclastic Sediments and Rocks. Geological Society of America Bulletin
Marti, J., & Ernst, G. (2005). Volcanoes and the Environment. Cambridge University Press.
Minakami, T. (1942). On the Distribution of Volcanic Ejecta. Bulletin of the Earthquake Research Institute, Tokyo.
Scott, W. E. (1989). Volcanic and Related Hazard. Vancouver, Washington: US Geolotical Survey. USGS. (2010, May 24). Volcanic Hazards. Retrieved June 13, 2013, from US Geological Survey (USGS): website