Seismic monitoring is critical for Long Valley, California. Long Valley is in a very tectonically active area, which makes it very susceptible to volcanism and high seismic activity. Long Valley has many places for vacationers and adventure sports enthusiasts, as well as several towns that are home to thousands of people. The Long Valley Caldera and surrounding areas are still considered active and must be monitored in order to prevent great destruction during an eruption. Beginning in the 1980s, many seismic stations were put in place all throughout the caldera and surrounding area to begin monitoring earthquake swarms that could be signaling an eruption. The data from the seismic stations, including location of events, depth of events, intensity of events, magnitude distribution of swarms, and magnitude of events, can be used to look for patterns that would separate the earthquake swarms from being a release of accumulated stress and being caused by a volcanic intrusion. Along with other volcanic monitoring of volcanic gas emissions and ground surface deformation, geologists are able to better determine the cause of earthquakes and if an eruption is looming in the near future. There have been some volcanic intrusion triggered swarms, which are usually accompanied by other signs of volcanism, as well as some swarms just from release of accumulated strain. By looking at the distribution and location of earthquake swarms and where is being emitted, Hill et al. and Hill & Prejean determined that there appears to be several dykes and sills below the surface of Mammoth Mountain and the south moat of the caldera that are allowing volcanic fluid to move closer to the surface (2006, 2005). It is not believed that there is a large magma chamber beneath either Mammoth Mountain or the caldera, and there is no real worry of a very large eruption in the very near future.
Seismic Monitoring and its Role in Tracking Volcanic Unrest and Seismicity in Long Valley and Mammoth Lakes, California
Seismic monitoring is crucial to Long Valley, California because Long Valley is located in a very volcanically, tectonically, and seismically active area of the world. There are great places to hike, ski, and bike all throughout the mountain ranges that flank the valley; all of which are home to thousands of people. For that reason alone it is critical that future volcanic eruptions and activity be predicted so that the communities nearby can be evacuated in time, and increased seismic activity is one sign of a possible eruption. Recently, geologists, seismologists, and volcanologists have begun to focus their efforts on understanding the history of Long Valley to predict the future. To do so, seismic monitoring has been improved upon and increased in order to create a record of seismicity in Long Valley and so changes can be noticed when trying to predict when a magmatic body might be moving closer to the surface.
The reason Long Valley is so tectonically, seismically, and volcanically active is because it is located near a transform/ normal fault boundary between the North American Plate, which Long Valley and the Sierra Nevada Mountains are on, and the Pacific Plate. The North American Plate is moving south-southeast, while the Pacific Plate is moving north-northwest. This plate movement is taking place because tension and shear forces are pulling against each of the plates. These forces are radiated through the plates causing there to be many smaller normal and transform faults, as well as the most common fault that is a combination of the two. Part of the western United States, beginning in the Sierra Nevada Mountains and ending in the Rocky Mountains, is the basin and range province, because these normal/ transform faults create repeated ranges and basins. The repeated normal faults create a rippled topography in the area. Also due to the tension forces the plate is being stretched and thinned, making it easier for volcanic fluid to affect the ground and water in the area. In Long Valley there are many smaller faults under and on the surface due to the tension and shear stress. These smaller faults, along with the larger normal faults in between the basins and ranges, create easy ways for magma to get closer to the surface (Hill, 2006).
One prominent feature of Long Valley is Long Valley Caldera. It is a product of the Long Valley eruption, which occurred about 760,000 years ago (Hamburger, 2010). The caldera is flanked by Mammoth Mountain on its southwest side, which is home to one of the most populous cities in Long Valley, Mammoth Lakes. Mammoth Lakes is located on the eastern flank of Mammoth Mountain. Its population can range in from 6000 to over 40,000 people (Hill & Prejean, 2005). It is home to a ski resort and many summer outdoor sports centers. Mammoth Mountain is a smaller dacitic volcano that is much younger than the caldera, having erupted about 57 thousand years ago (Hill, 2006). Though in human time these eruptions both occurred a long time ago, in geologic time they occurred relatively recently and have the potential to erupt sometime in the near geologic future. It is very important to monitor the behavior of the area in order to prevent death and great destruction from an eruption. Some signs of a looming eruption are increased seismic activity, release of volcanic gases from under the surface, and deformation, in this case, in the form of a resurgent dome. For the most part, this paper will focus on the monitoring of seismic activity in Long Valley, but connections will be made between all three signs. One sign is not enough to say an eruption is going to occur, but an organized combination of all three is pretty sufficient to determine if one is likely.
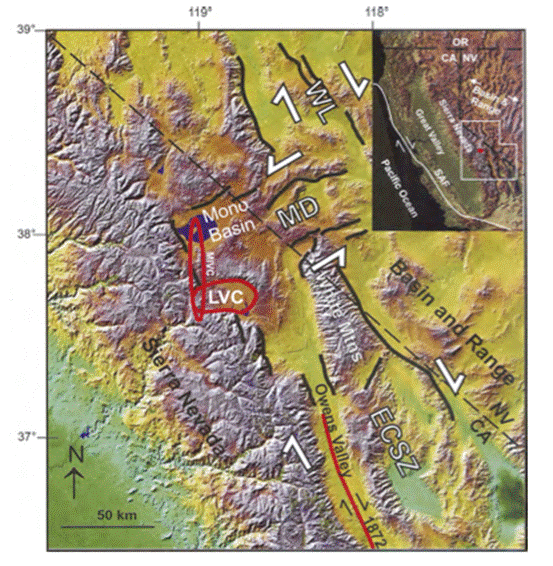

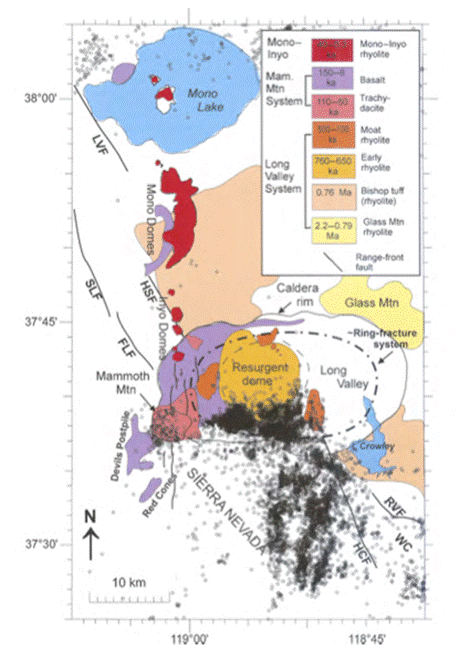
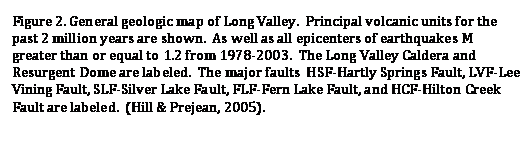
Seismic monitoring is extremely important in Long Valley. It can be used to track normal seismicity of the area, earthquakes caused by built up strain being released, and seismic activity due to magmatic fluid moving beneath the surface. All three are important when dealing with a volcanically active area because the normal seismicity must be known for changes to be noticed, earthquakes that are the result of strain being released can trigger eruptions in delicate settings like Long Valley, and earthquakes caused from magmatic movement may be signifying a looming eruption. Over the last four decades seismic monitoring has been increased and improved in Long Valley. The increase in seismic monitoring is helping to create a record of the “normal” seismicity of Long Valley. Since the seismic monitoring really began around 1980, there have been several periods of a lot of seismic activity and periods of almost no activity (Hill et al., 2003). The records have not been kept long enough for the normal to be determined. It could be that the normal is back and forth periods of activity and quiet. All of the episodes of earthquake swarms have pretty much been consolidated on the south central to south west side of the caldera. The swarms seem to range from beneath Mammoth Mountain, in the south central part of the caldera, and along the south moat of the caldera near Convict Lake, also known as the South Moat Seismic Zone (SMSZ) (Hill et al., 2003). This caused initial concern that an eruption may be coming in the near future from either Mammoth Mountain or the southern part of the caldera. Also right around the same time, an area in the southwestern center of the caldera began to rise; this area is known as the resurgent dome. The earthquake swarm and resurgent dome locations can be seen in Figure 2. About 8 years after the 1980 unrest, magmatic
began to be emitted from the ground on Mammoth Mountain (Hill & Prejean, 2005). All of these being signs of movement of volcanic fluid under the surface makes scientists wonder about a future eruption even more. However, no eruption has occurred yet after almost 30 years of recorded unrest. To further explore this; seismic monitoring can be used to look into the behavior of the individual earthquake swarms. The location, migration, and magnitude distribution of the earthquakes can all be used to determine the cause of the earthquake swarms.
In order to begin creating a record of seismic activity and begin analyzing the seismicity of the area, many more seismometers had to be set out in Long Valley. In the late 1970s and early 1980s the number of seismometers within 10 km of the caldera went from about 3 to nearly 20 (Hill et al., 2003). Most of the seismometers deployed are short-period, vertical component seismometers (Hill et al., 2003). These seismometers are constantly recording the movement of the earth in the form of seismograms. Figure 3 is a map of the seismometers in the caldera and the SMSZ. The seismometers were placed based on location and range in comparison to the area of interest. It is necessary to have three seismometers if not more to find an accurate epicenter. It’s useful to have some seismometers close to the area of interest to catch smaller magnitude earthquakes and to have some seismometers further away so that when larger magnitude events occur the seismogram is readable. Also sometimes seismometers will not record an event because it may be too small or the seismometer may have been down, so it is always good to have numerous seismometers in an area.
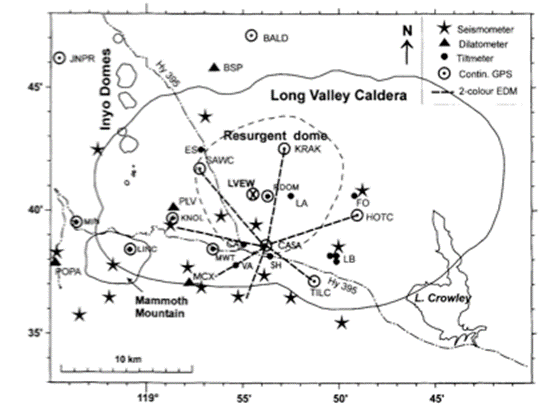
Figure 3. Seismic Monitoring Stations in place in 1999. (Hill et al., 2003).
The data taken from the seismic stations can then be used to do many different things. The seismographs will record the frequency and amplitude of the wave as well as the time of the primary (P) and secondary (S) waves. A simple way to calculate magnitudes for a swarm of earthquakes with a lot of small magnitudes is to calibrate each of the smaller events to some of the larger events with well-known magnitudes using the largest amplitudes of each seismogram, for each event. The equation, (individual event magnitude)= log[(individual event amplitude) /(calibration event amplitude)]+(calibration event magnitude), is the equation used to calculate magnitude. The calibration event amplitude and the individual event amplitudes must be from the same station for them to be accurate. This is a very simple way to calculate the magnitudes of smaller events. Once magnitude is determined a lot of analysis can begin. For the swarms that have occurred, the distribution of small and large magnitudes is very important when trying to decide the cause of the earthquakes. This is because an earthquake swarm caused by movement of magmatic fluid typically would have a very high b-value, meaning it is rich with small magnitude earthquakes (Hill et al., 2003). The equation
, where N is the cumulative number of earthquakes greater than a given magnitude M, and A is a constant, can be used to find b (Hill et al., 2003). However, a high b-value does not always mean that magmatic movement is the cause of the earthquakes. If an earthquake swarm has a high b-value it could be used as a second or third piece of evidence pointing towards a future eruption, but not as a solitary reason to believe an eruption will occur. If b-values for one or more swarms of earthquakes have been determined, the next thing that can be done is to look at other phenomena that are occurring in the areas in and around where the swarms are occurring.
There are three swarms that create a lot of interest because of their coincidence with other phenomena and are commonly classified as periods of unrest; the 1978-1983 swarms, the 1989 11 month swarm in Mammoth, and the 1997-98 swarms. The 1978-1983 swarms coincided with the largest uplift of the resurgent dome. From 1978-1983 the resurgent dome increased by up to 80 cm (Hill et al., 2003). Also there were four earthquakes of a magnitude equal to or greater than 6 in 1980, the first occurring near Convict Lake, the second in the south moat, the third near the south edge of the caldera, and the fourth a few days later about 10 km south of the caldera (Hill, 2006). These earthquakes caused the citizens and officials of Mammoth Lakes to begin to worry and plan evacuation routes. An escape route was created so that there would be more than one way off the mountain in case of an eruption (Wikipedia, 2010). There were also two magnitude 5.3 earthquakes that occurred in 1983 that were accompanied with dome movement. Hill et al. determined that the b-value of this swarm was ~0.9, which is considered to be low (2003). Despite the low b-value, Hill et al. along with many other papers decided that 1978-1983 was a period of volcanic unrest because of the concurrence of the earthquake swarms and dome movement (2003).
In 1989 an 11-month series of earthquakes began, as well as a series of long-period deep earthquakes and CO2 emission on the flanks of Mammoth Mountain. Both the long period earthquakes and the
emission continued on past 2005. Hill & Prejean determined the b-value of this swarm to be ~1.17, which is relatively high (2005). A good amount of the earthquakes in this swarm were concentrated under Mammoth Mountain. The long-period earthquakes and the
emission were under and on Mammoth Mountain as well. Both Hill et al. and Hill & Prejean concluded that the 1989 swarm was due to a basaltic intrusion under Mammoth Mountain (2003, 2005). A very detailed analysis of how this was determined is in Hill & Prejean, 2005. In Figure 4 the depth, longitude, and latitude of each earthquake is plotted against time, so the movement of the earthquakes over the 11 months can be seen. Hill & Prejean also concluded that the 1989 swarms were triggered by the release of
from dykes and sills at mid-crustal depths (2005). The 1989 swarms are an example of when the b-value was used as supporting evidence for a possible magmatic intrusion.
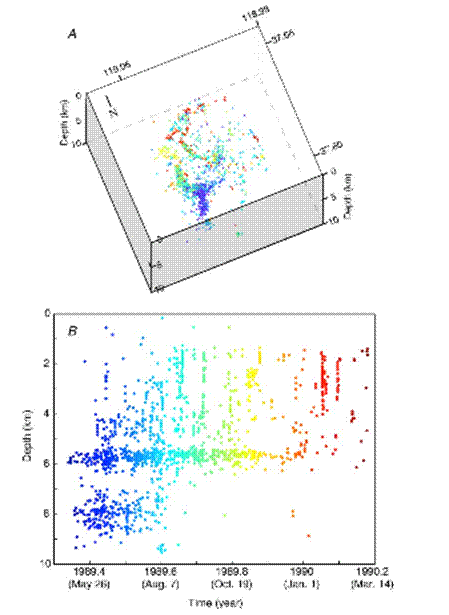
Figure 8. High-resolution hypocentral locations for brittle-failure earthquakes in the 1989 swarm (Hill & Prejean, 2005). (A) Three-dimensional perspective view looking down to the northeast (N238E). Elevation of view is 76 0 from the horizontal. (B) Time color-code keyed to plot of focal depths with time.
The 1997-98 unrest consisted of a rising of the resurgent dome then a swarm of earthquakes along with more rising of the resurgent dome. Hill et al concluded that the 1997-98 swarms were caused by a magmatic intrusion rather than release of accumulated stress because of the coincidence of the deformation and the swarms. The resurgent dome had not really uplifted very much since the 1980s. The 1997-98 uplift was the second greatest right behind the 1980 uplift. Also it had a relatively high b-value of ~1.2 (Hill et al., 2003). There were swarms both in 1996 and 1999, but they were determined to be earthquakes from release of accumulated strain because there was no evidence that magma was being transported beneath the surface and they both had very low b-values. This is an example of when the b-value can be used to support other evidence that a swarm is not from volcanic intrusion.
A lot is still unknown about Long Valley, the caldera, and Mammoth Mountain, but a great amount of progress has been made in learning about their histories and their futures. Most scientists don’t think that there is a large magma chamber beneath either the caldera or Mammoth Mountain. Hill & Prejean and Hill et al. both suggest that there are series of dykes and sills beneath both, that were caused by the faulting of basin and range, that are allowing magma to rise up closer to the surface (2005, 2003). This causes
to be emitted, the land of the resurgent dome to rise up slightly, and the rock to move creating earthquake swarms. This was hypothesized because the mapping of the depths and locations of earthquakes can show where a magma chamber might be, as Hill & Prejean did (2005). Also being able to determine the b-value can be used as supporting evidence for volcanic intrusion. Both of which would not be possible with out seismic monitoring. The knowledge that has been gained and that will be gained from seismic monitoring of Long Valley could save thousands of lives in the near future and if anything it will provide more understanding into other calderas and their behavior.
References Cited
Hamburger, Michael. Sierra Nevada G190 Class Lecture. Indiana University. May
2010.
Hill, D.P. 2006. Unrest in Long Valley Caldera, California, 1978-2004, in Mechanisms
of Activity and Unrest at Large Calderas, edited by D. Troise et al., Geological
Society Special Publication, 269, 1-24.
Hill, D.P., Langbein, J.O. & Prejean, S. 2003. Relations between seismicity and
deformation during unrest in Long Valley Caldera, California, from 1995
through 1999. Journal of Volcanology and Geothermal Research, 127, 175-193.
Hill, D. P. & Prejean, S. 2005. Volcanic Unrest Beneath Mammoth Mtn., California.
Journal of Volcanology and Geothermal Research, 146, 257-283.
Long Valley Caldera. (5 June 2010). In Wikipedia, the free encyclopedia. Retrieved
June 8, 2010, from http://en.wikipedia.org/wiki/Long_Valley_Caldera
Prejean, S., Ellsworth, W., Zoback, M. & Walhouser, F. 2002. Fault structure and
kinematics of the Long Valley Caldera region, California, revealed by high-
accuracy earthquake hypocenters and focal mechanism stress inversion. Journal of Geophysical Research, 107(B12), 2355, doi: 10.1029JB001168.