More than 100 alpine glaciers are found in the Sierra Nevada Range. The Sierra Nevada region typically experiences hot, dry summers and cold, wet winters. These atmospheric conditions allow for heavy snowfall at high altitudes and result in the formation of glaciers in the Sierra Nevada Mountains. Scientists believe that the glaciers found in California today were formed about 700 years ago during the Little Ice Age (1250-1900 A.D.). Glaciers are a significant part of the historical geology of California because they altered the regional landscape with a variety of erosional and depositional landforms. Changes in the size of Sierra Nevada glaciers have been observed for the last 150 years. The purpose of this paper is to investigate how glaciers in the Sierra Nevada region have changed in size over time and discover if there is a relationship between glacier behavior and local climate. It has been determined that during the 20th century, 14 Sierra Nevada glaciers measured a combined decrease in area of 2.14 km² ± 0.10 km. During this same time period, the mean surface air temperature of the Sierra Nevada region increased by approximately 0.6 ° C, while mean winter precipitation decreased by 14.9 mm. Consequently, there appears to be a correlation between warmer, drier climate conditions and glacier retreat in the Sierra Nevada region during the 20th century. Many glaciers around the world have also decreased in size as global temperatures have increased by 0.74 ° C in the last century. Glaciers are an important part of the hydrologic cycle and alpine ecosystems, and monitoring their state of health needs to be continued.
Introduction
People from around the world travel to the Sierra Nevada region in California to witness the majestic beauty of the glaciers and the glacial landforms present there. More than 100 alpine glaciers are found in the Sierra Nevada Range at latitudes between 36.37° and 38.37° North (Basagic, 2008). These glaciers range in elevation from 3090 to 4148 meters and occupy an area of 15.87± 1.69 km² [Raub et al. (2006), as reported by Basagic (2008)]. The Sierra Nevada Mountains, located in the eastern part of the state, are 640 km in length and vary in width from 65 to 130 km (Basagic, 2008). The north to south orientation of this mountain range allows large amounts of orographic snow accumulation as moist air from the Pacific Ocean moves eastward towards the mountains in the winter months (Guyton, 1998). The Sierra Nevada region typically experiences hot, dry summers and cold, wet winters [NCDC (2006), as reported by Basagic (2008)]. These atmospheric conditions allow for heavy snowfall at high altitudes and result in the formation of glaciers in the Sierra Nevada Mountains (Figure 1).
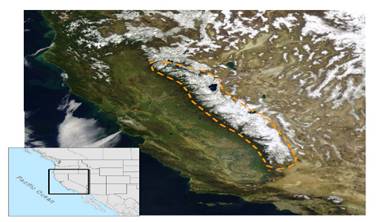
Figure 1: Figure reproduced from Basagic [2008] (exact reproduction). This is a NASA MODIS image from March 3, 2004 showing the geography of the Sierra Nevada Range in North America.
Glacial History of the Sierra Nevada Region
In the 4600 million year history of the Earth, there have been a series of glacial and interglacial periods (Hambrey and Alean, 2004). Cooler time periods when glaciers covered large areas of Earth’s continents were followed by decreased glacier coverage during warmer time periods. The most recent Ice Age occurred during the Pleistocene Epoch which began 1.6 million years ago and ended approximately 10,000 years ago (Guyton, 1998). Glaciers are a significant part of the historical geology of California. Geologic evidence indicates that five major glacial advances occurred in the Sierra Nevada region during the Pleistocene Epoch and include: McGee, Sherwin, Tahoe, Tioga, and Recess Peak [Blackwelder (1931), Bailey et al. (1990), and Clark and Gillespie (1997), as reported by Basagic (2010)]. In between these periods of glacial advance, many of the Sierra Nevada glaciers retreated or even disappeared (Guyton, 1998). It appears that when the Pleistocene Epoch ended, an interglacial period of warming temperatures occurred which resulted in the temporary disappearance of glaciers in California (Guyton, 1998).
During the late Holocene Epoch, several periods of glacial advance occurred worldwide. Geologic evidence indicates that glacial maxima occurred approximately 2200, 1600, 700, and 170-250 years B.P. [Bowerman (2006), as reported by Basagic (2008)]. Scientists believe that the glaciers found in California today were formed about 700 years ago during the Little Ice Age (Guyton, 1998). The Little Ice Age (LIA) refers to a cooler time period from 1250-1900 A.D. when glaciers in the northen hemisphere typically increased in area (Guyton, 1988). Moraine, tree-ring, and lake sediment data collected in the Sierra Nevada region indicate the prevalence of cool climate conditions during the LIA which allowed glaciers to advance [Stine (1996), as reported by Basagic (2008)]. Based on dendochronology and photographic records from the Sierra Nevada region, Curry (1969) concluded that the most recent glacial maxima occurred between 1895 and 1897 (Basagic, 2008). In the 20th century, however, periods of retreat were more common than advance for many glaciers around the world (Hambrey and Alean, 2004).
Past Scientific Study of the Sierra Nevada Glaciers
Glaciers are often difficult to study because they are located in remote locations which are not easily accessible. As a result, not much information was known about the Sierra Nevada glaciers until the last 150 years. In 1871, Clarence King was the first person to publish an account indicating that alpine glaciers could be found in the western United States (Guyton, 1988). The following year, John Muir conducted velocity studies on Maclure and Lyell Glaciers which confirmed these two Sierra Nevada glaciers were “living glaciers” (Guyton, 2008). In 1930, François Matthes proposed the concept that erosion by both rivers and glaciers shaped the Yosemite Valley (Guyton, 1988). The National Park Service (NPS) began to document glacier changes at Yosemite National Park when near annual glacier surveys were started in 1931 (Basagic, 2008). The U.S. Geological Survey, the California Division of Mines and Geology, and numerous university researchers have also been involved in long term monitoring of the Sierra Nevada glaciers (Guyton, 1988). These past scientific studies have allowed greater understanding of the glaciers of the Sierra Nevada Range.
Glacier Characteristics
Glacier Definition
Exactly what is a glacier? A glacier is defined as a perennial mass of ice that flows downhill like a thick fluid (Granshaw and Fountain, 2010). This body of ice flows from areas of higher altitude to lower altitude as a result of its own weight. A more detailed definition provided by Raub et al. (2006) specifies that a glacier must occupy a minimum area of 0.01 km² and exhibit one or more of the following characteristics: “(1) snow or ice accumulated over several years, (2) a crevasse, (3) heavily debris-covered ice which exhibits evidence of flow, and (4) moraine or trim lines” (Basagic, 2008). Ice patches, snowdrifts, snowfields, and snow patches would therefore not fit the definition of a “true” glacier, but would be considered perennial snow masses (Basagic, 2008). Glaciers are found in areas of high latitude, high altitude, or areas of both high latitude and high elevation. In order for a glacier to move, the mass of ice must be a certain size. On average, a mass of ice begins to flow downhill when its thickness is a minimum of 120 feet (Guyton, 1988). Also, the critical shear stress at the bottom of a glacier must exceed 10⁵ Pa which allows the ice to deform, become plastic, and move downhill [Paterson (1999), as reported by Basagic, 2008)]. Glaciers are in constant motion because this ice flows under the influence of gravity.
Zones of a Glacier
Mountain glaciers can form in areas where the amount of snowfall exceeds the amount of snow that melts. The accumulation zone of a glacier is located at higher altitudes and is snow covered year round (Granshaw and Fountain, 2010). In the accumulation zone, snow falls and compresses older snow beneath it and these snow crystals compact and recrystallize to form denser ice (Figure 2). There are three layers of the accumulation zone (Granshaw and Fountain, 2010). The upper layer consists of light snow, the middle layer (firn) is a transitional state between snow and dense ice, and the bottom layer consists of dense ice (Granshaw and Fountain, 2010). As snow is converted to ice, air is forced out of the ice crystals and the density increases from 0.1 for snow to 0.9 for ice (Guyton, 1988).
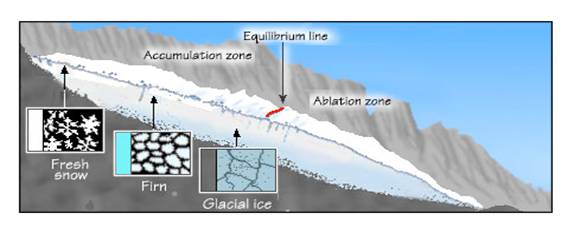
Figure 2: Figure reproduced from Granshaw and Fountain [2010] (exact reproduction). This cross section of a glacier shows the zones of a glacier and the different crystal structures of snow and ice present in a glacier.
The ablation zone of a glacier is found in the lower altitudes of a glacier where more snow melts, evaporates, or sublimates than accumulates (Hambrey and Alean, 2004). Ablation, therefore, involves a net loss of ice. Conditions that can affect the rate of ablation on the surface of a glacier include: slope aspect, humidity, wind, cloudiness, shadiness, amount of solar and terrestrial radiation, and amount of melt-water percolation (Owen et al., 2009).
The equilibrium line of a glacier is a transitional area where the amount of accumulation equals ablation (Granshaw and Fountain, 2010). During the summer, the equilibrium line is the boundary between the snow covered upper portion of the glacier and the ice-exposed lower part (Granshaw and Fountain, 2010). The location of the equilibrium line on a glacier is influenced by local climate conditions (Granshaw and Fountain, 2010). During warmer years, the equilibrium line tends to move to higher altitudes, while during cooler years the equilibrium line tends to move to lower altitudes. This change in position of the equilibrium line to higher or lower altitudes indicates either glacier advance or retreat (Granshaw and Fountain, 2010). Glaciers advance when the amount of ice flow downhill is greater than the amount of snowmelt at the terminus of a glacier (Tarbuck and Lutgens, 2003). On the other hand, glaciers retreat when either snowfall decreases or snowmelt increases and the terminus of the glacier moves further uphill (Guyton, 1988).
Mass Balance of a Glacier
The mass balance of a glacier is a year by year measure of the balance, or lack of balance, between snow accumulation and ablation of a glacier (Hambrey and Alean, 2004). Comparing the accumulation zone area to the ablation zone area gives an indication of the health of a glacier; the larger the accumulation zone size, the healthier the glacier (Guyton, 1988). A glacier in a balanced state of equilibrium has a stationary terminus (Tarbuck and Lutgens, 2003). Positive mass balance of a glacier occurs when snow accumulation exceeds ablation and the glacier terminus will continue to advance downslope until the mass gained by accumulation equals the mass lost by ablation (Pelto, 2010). A negative mass balance occurs when a glacier is in a state of disequilibrium where ablation exceeds accumulation for a particular year (Hambrey and Alean, 2004). When a glacier retreats, its zone of ablation decreases in size until a new balance is reached between accumulation and ablation and the glacier terminus then becomes stationary (Tarbuck and Lutgens, 2003). Small valley glaciers respond more quickly to changes in mass balance, while large glaciers may take several decades to see visible changes (Hambrey and Alean, 2004).
To measure the mass balance of a glacier, stakes are placed at various locations on the accumulation and ablation zones of a glacier (Hambrey and Alean, 2004). The elevation of the glacier surface at different points is measured at the onset and end of the ablation season and the movement of these stakes is used to calculate ice velocities of the glacier as it moves downhill. This data can be used to calculate changes in water mass and glacial volume (Hambrey and Alean, 2004). Mass balance measurements can therefore be used to evaluate glacier changes.
Glaciers Alter the Regional Landscape
Alpine glaciers are powerful agents of erosion because sediment and rocks which are located underneath the glacier are dragged along with the moving ice. Consequently, the bedrock is scratched and scraped as the glacier moves downhill. Glacial erosion alters the regional landscape and results in the formation of landforms such as horns, arêtes, cirques, and U-shaped valleys (Owen et al., 2009). As glaciers move across the landscape, they also deposit ridges of sediment known as moraines (Owen et al., 2009). A moraine is a pile of till which is composed of poorly sorted sediment such as sand, gravel, and boulders (Guyton, 1988). Lateral moraines form along the sides of a glacier, while a terminal moraine forms near the end of a glacier (Granshaw, 2010). Terminal moraines are often used to determine changes in glacier area over time because they mark the outline of glacier position at an earlier time (Owen et al., 2009). Radioactive isotope dating of
or
present in rocks found in till can be used to determine information about past glacier position (Owen et al., 2009).
Recent Sierra Nevada Glacier Changes
Changes in the size of Sierra Nevada glaciers have been observed by scientists for many years. In 1942, F.E. Mathes provided evidence that Lyell Glacier retreated 87 feet between 1931 and 1939 (Guyton, 1988). According to N. King Huber, Dana Glacier lost 75% of its surface area between 1908 and 1975 (Guyton, 1988). More recently, Basagic (2008) conducted a research study in which he evaluated 14 Sierra Nevada glaciers. Since some these glaciers are small and currently unnamed, he gave them informal names based on their proximity to nearby mountains (Table 1).
Table 1: Sierra Nevada Glaciers Studied by Basagic (2008). Table reproduced from Basagic [2008] (exact reproduction). It provides location, size, elevation, steepness, and orientation of the study glaciers. Elv=elevation (meters), Slp=slope (°), Asp= aspect (º).
Basagic (2008) used photographic records, geological records,moraine data, and topographic maps from previous years and compared this with current information to evaluate glacier changes in the Sierra Nevada during the 20th century. Basagic (2008) determined that these 14 Sierra Nevada glaciers decreased in combined area by 2.14 km² ± 0.10 km² over the last century with an average loss of 0.15 km² for each glacier. On average, these glaciers decreased in area by 55% since 1900 (Basagic, 2008). A comparison between the surface area and fractional area loss of each glacier between 1900 and 2004 is presented in Figure 3. East Lyell Glacier experienced the greatest fractional area loss of 78%, while Lilliput Glacier experienced the lowest fractional area loss of 31% (Basagic, 2008).
Figure 3 : Figure reproduced from Bassagic [2008] (exact reproduction).This graph represents changes in surface area and fractional area in 14 Sierra Nevada glaciers. The black bars represent glacier area in 1900, while the white bars represent glacier area in 2004. The open squares represent fractional area loss, and uncertainty is represented by error bars.
According to Basagic (2008), rapid retreat of the Sierra Nevada glaciers occurred in the first half of the 20th century followed by a slower rate of retreat from the 1950’s through the early 1980’s. In the 1980’s and early 1990’s the rate of retreat stabilized, but this was followed by a rapid rate of retreat in the late 1990’s (Basagic, 2008). Although some periods of glacier advance in the Sierra Nevada Mountains were seen during the 20th century, glacial retreat was more prevalent.
How Climate Affects Glaciers
The local climate of an area can affect the size of a glacier. Glaciers respond to changes in air temperature and snowfall. Glaciers tend to increase in area when winter precipitation is above average and summer air temperatures are lower than average, and decrease in area when these conditions are reversed (Granshaw and Fountain, 2010). Summer surface air temperatures determine the amount of snowmelt, while winter air temperatures determine whether the precipitation accumulates as snow or rain (Basagic, 2008). Glaciers typically advance in cool, wet years because snow and ice accumulate, while during warm, dry years glaciers will retreat because more snow melts than accumulates (Granshaw and Fountain, 2010). The mass of glaciers will consequently change as glaciers advance and retreat.
Recent Climate Trends in the Sierra Nevada Region and the Effect on Local Glaciers
Since the local climate of an area has an affect on glacier behavior, it is an important variable to look at when studying glacial changes. By comparing monthly temperature data from 1885 to 2004, Basagic (2008) determined that the mean surface air temperature in the Sierra Nevada region has increased by approximately 0.6° C in the last century. Overall, warmer annual temperatures were observed in the Sierra Nevada region from the mid 1920’s through the mid 1940’s (Figure 4). Excluding a warming period in the early 1960’s, cooler periods were observed during the mid 1940’s through the early 1990’s (Basagic, 2008). A warming period occurred from the mid 1990’s to the beginning of the 21st century. Although there were some periods of cooler temperature during the 20th century, there was a strong increase in mean annual temperature from the mid 1990’s to 2004 (Basagic, 2008). 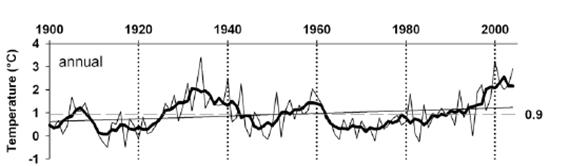
Figure 4: This figure is adapted from Basagic [2008] (modified version). Mean annual temperature of the Sierra Nevada region from 1900-2004 is represented by grey lines, trend line for 5 year running mean is the thick black line, and mean seasonal temperature is the dashed grey line.
The amount of precipitation an area receives is another variable which affects the size of a glacier. During the 20th century, the mean winter precipitation of the Sierra Nevada region decreased by 14.9 mm (Basagic, 2008). Along with warmer temperatures which increased the amount of snow melt, there was also an overall decrease in the amount of snow which fell on the Sierra Nevada region.
The delicate balance between glacial accumulation and ablation is very sensitive to climate change (Owen et al., 2009). Basagic (2008) determined that as the local climate became warmer and drier during the 1920’s to the 1950’s, the Sierra Nevada glaciers retreated. Overall, when the climate became cooler and wetter during the 1950’s through the 1980’s, the Sierra Nevada glaciers advanced (Basagic, 2008). In the late 1980’s to the beginning of the 21st century, glacier retreat again occurred as warmer climate conditions prevailed (Basagic, 2008). The data suggest there appears to be a correlation between climate and glacier change in the Sierra Nevada region.
How Climate Has Affected Glaciers Worldwide
Similar to the overall warming trend seen in the Sierra Nevada region during the 20th century, the mean annual worldwide surface air temperature has increased 0.74° C
± 0.18°C in the last 100 years [Trenberth et al. (2007), as reported by Basagic( 2008)].
Even though there is variability in glacier response as a result of differences in regional temperatures and precipitation levels, loss of glacier area has occurred worldwide in the last century. Like the glaciers of the Sierra Nevada region, other glaciers in the western United States and around the world have also retreated (Figure 5). Columbia Glacier in Alaska has retreated by 134 m since 1984, and glaciers in Glacier National Park have lost 73% of their area from 1850 to 1993 (Pelto, 2010). Glaciers in the European Alps have decreased in area by 35% since 1850 (Basagic, 2008).The Kilimanjaro Ice Cap in central Africa has experienced an 82% loss of area since 1912 (Hambrey and Alean, 2004). Many of the glaciers located in tropical mountains are in danger of disappearing within the next few decades (Hambrey and Alean, 2004).
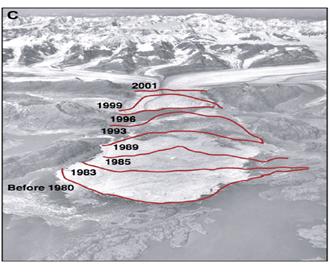
Figure 5 Figure reproduced from Owen et al. [2009] (exact reproduction). This glacier (Columbia Glacier of Alaska) is a classic example depicting how many glaciers have retreated in the last 30 years.
The World Glacier Monitoring Service annually compiles data from 7 glaciers in the southern hemisphere and 76 glaciers in the northern hemisphere and has determined that the mean mass balance for glaciers worldwide has been negative from 1991 through 2008 (Pelto, 2010). Mean decadal mass balance loss increased from 200 mm. w.e. (water equivalent) in the 1980’s to over 600 mm w.e. in the 2000’s for glaciers worldwide (Figure 6). As indicated by this data, many glaciers around the world are losing mass and this may lead to widespread glacial retreat.
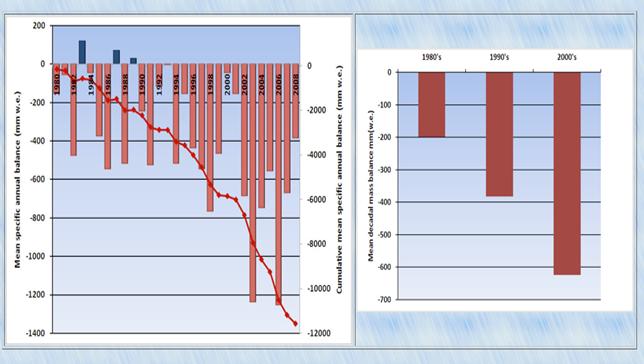
Figure 6: Figure reproduced from Pelto [2010] (exact reproduction).This graph represents data from the World Glacier Monitoring Service, which shows the change in world glacial mass balance from 1980 to 2008. Bars above the zero mass balance line indicate positive mass balance, while bars below this line indicate negative mass balance. (w.e. =water equivalent).
Environmental Effects of Glacier Recession
Glacier Recession Affects the Hydrologic Cycle
Glaciers are important in the western United States because this area typically has dry summers and glacier melt-water buffers streams which decreases the occurrence of drought and contributes to local river discharge (Guyton, 1998). For example, Lyell and Maclure Glaciers which are both located in Yosemite National Park contribute to the Tuolomne River (Basagic, 2008). A decrease in glacier area leads to drier conditions in nearby ecosystems as a result of a reduction in the amount of glacial runoff. As a result, glacial recession has an effect on ecoystems because it alters the hydrologic cycle. Animals and plants depend on glacial melt-water for their survival. Humans also rely on glacial melt-water for irrigation purposes, for hydroelectricity generation, and as a source of drinking water (Hambrey and Alean, 2004). Consequently, large populations of people can be affected by glacier changes.
Glacier Recession Increases Sea Levels
The melting of glaciers worldwide has led to a rise in global sea level and an increase in ocean volume. Data indicates that more than 50% of the current rate of 3.1 mm/year of sea level rise can be attributed to the loss of glacier area (Owen et al., 2009). It appears that human actions may have contributed to the loss of glacier area in the last century due to fossil fuel consumption and deforestation which have led to increased CO2 levels in the atmosphere (Hambrey and Alean, 2004; Guyton, 1988). According to a 2001 report from the Intergovernmental Panel on Climate Change (IPCC), global temperatures are expected to increase 1.4-5.8° C as a result of increased CO2 emissions by 2100 A.D. (Hambrey and Alean, 2004). The U.S.G.S. estimates that if all the world’s mountain glaciers melted, sea levels would rise by 0.5 m which could lead to flooding of many low lying areas of the world (Hambrey and Alean, 2004).
The Future of Glaciers
Climate variables appear to be a factor in recent glacier changes. Because alpine glaciers occur only under specific climate conditions, they are sensitive to environmental changes in temperature and precipitation. Since the Little Ice Age, mountain glacier retreat has been more prevalent than glacial advance (Hambrey and Alean, 2004). It is predicted that many tropical glaciers will disappear by 2020 and approximately 50% of the current mountain glacier mass will be lost by 2100 (Hambrey and Alean, 2004). Specifically looking at the Sierra Nevada glaciers, data suggest these glaciers have decreased in size as a result of warmer local temperatures which have increased an average of 0.6°C in the last century. According to Basagic’s (2008) observations of 14 Sierra Nevada glaciers, using the current mean retreat rate of 0.0012 km² per year, these glaciers are predicted to disappear within the next 250 years ± 200 years given constant conditions. This pattern of glacial retreat seen in the Sierra Nevada region is similar to that exhibited by glaciers worldwide. It appears that rising global temperatures have had an effect on the size of glaciers in the Sierra Nevada region and around the world.
The recent trend of the sustained negative mass balance of Earth’s glaciers is a complex issue. Although in Earth’s history there have been natural variations in climate which have caused glacial and interglacial periods, it appears that human actions as a result of industrialization may have contributed to increased global temperatures and subsequent loss of glacier mass. Further study of this problem needs to be continued because glaciers are an important part of alpine ecosystems as well as the hydrologic cycle.
References Cited
Basagic, H. J. IV. 2008. Quantifying twentieth century glacier change in the Sierra
Nevada, California. Master’s thesis, Portland State University: 1-114.
Basagic, H. J. IV. 2010. Glaciers of the American West: Glaciers of California.
Portland State University. Retrieved from http://glaciers.research.pdx.edu.html.
Granshaw, F. D. & A. G. Fountain. 2010. Glaciers and glacier change: Glacier
change in the Upper Skagit River Basin. Retrieved fromhttp://www.glaciers.pdx.edu/Projects/LearnAboutGlaciers/Skagit/index.html.
Guyton, B. 1998. Glaciers of California. Berkeley, CA: University of California Press.
Hambrey, M., & J. Alean. 2004. Glaciers. 2nd ed. Cambridge, U.K.: Cambridge
University Press.
Owen, L. A., Thackray, G., Anderson, R.S., Briner, J., Kaufman, D.,
Roe, G., Pfeffer,W. & C. Yi. 2009. Integrated research on mountain glaciers: Current status, priorities and future prospects. Geomorphology 103: 158-171. ScienceDirect.
Pelto, M. S. 2010. North Cascade Glacier Climate Project. Nichols College.
Retrieved from http://www.nichols.edu/departments/glacier.html.
Tarbuck, E. J. & F. K. Lutgens. 2003.Earth Science. 10th ed. Upper Saddle River, N.J.: Pearson Education, Inc.