Electrical power generated by geothermal energy is unique to tectonically active regions of the world. In places like the western United States, volcanism provides a strong geothermal gradient and efficient geothermal energy production. Steam-driven or binary geothermal power plants harvest the earth’s internal energy and convert it to electricity. The process is not altogether complicated but clever: superheated ground waters lying above magmas are pumped into a power plant; steam or a heated, highly pressurized hydrocarbon gas turns a turbine; and the turbine creates electrical power through a generator. However, compared to that of coal fuel technology, geothermal energy is a much smaller scale production. Geothermal energy from the earth may also be harvested in individual homes or businesses for heating and cooling regulation. There are improvements to be made and environmental concerns to be monitored, but this ingenious type of energy production is still considered low-impact, green energy.
Introduction
Over the past half-century, use of geothermal energy, a natural resource from deep in the earth, has boomed. Along with contributing a clean power source to their surrounding communities, geothermal power plants have found themselves in a battle to contribute to the nation’s power grid, maintain a healthy relationship with the public, and preserve the environment. Geothermal energy is a resourceful way to generate electricity, but it is not yet an adequate way to provide electricity for many largely populated areas. The importance of researching and improving this field lies within the energy crisis. Does geothermal energy offer a real solution?
Geothermal Energy
Around the globe, volcanism is a geologic process that allows for the production of geothermal energy. Movement of the earth’s crust and hot rock interaction with groundwater create a unique setting that allows humans to harvest the earth’s internal thermal energy. However, few regions of the world have ideal settings to utilize this natural process efficiently.
Geologic Setting
Volcanism is prevalent in areas there the earth’s crust is stretched or compressed, called divergence and convergence respectively. In a divergent zone, crustal plates or intra-plates are pulled apart by tensional forces. The thinning of this crust allows less-dense magmas from deep in the earth to rise up to the surface. In a convergent zone, crustal plates or intra-plates are pushed together, often on top of one another. This grinding and subduction of crust creates a high-heat melting atmosphere for rock in the foreland, and this melted less-dense rock, too, rises up to the surface. Plates may also experience friction and melting of rock along transform boundaries where the plates slide past one another. Magmas often rise to the surface in cracks or thin sections of crust called vents.
Along and around such faults where this tectonic activity occurs, a significantly higher geothermal gradient is created compared to that of tectonically inactive intra-plate areas. A geothermal gradient is the rate at which temperature increases with depth below the earth’s surface [Nelson, 2002]. The figures below demonstrate a geothermal gradient and areas of the United States that have relatively high underground temperatures, for example in the West. This region is known for its active tectonics, which is important in utilizing geothermal energy.
Figure 2. [Nelson, 2002] This diagram illustrates variations of geothermal gradients. An increase of about 25℃ per kilometer is the average gradient.
Figure 3. [Geothermal Technologies, 2006] This map shows estimated average temperatures 6 kilometers below the surface across the United States. Red areas represent strong geothermal gradients.
Water/Rock Interaction:
The hydrologic and geologic cycles interact to create “geothermal reservoirs,” an important coefficient in the energy production equation [Geothermal Information, 2010]. Rainwater and other surface waters infiltrate soil and bedrock, sinking far beneath the surface closer to the “hot rock.” In an area with an extreme geothermal gradient, such as in the West, groundwater is superheated to temperatures well above boiling point. Water temperatures can be anywhere from 100-350℃, yet the pressure of the overlying rock keeps the water in liquid form rather than allowing it to convert to steam or vapor [Wagner]. Sometimes, however, steam vents or cracks in the ground called fumaroles do allow for steam to escape the geothermal reservoir. These are apparent at the surface near geothermal power plants, but they are ephemeral and not induced by plant construction.
The convective patterns of the hot (and cold) water underground is complicated and still not thoroughly understood, so frequently drilling for hot water is unsuccessful. Layers of stacked hot and cold waters are often present, or sometimes the reservoir is not as large as originally thought. This makes drilling a very expensive venture that must be well-planned. When drilling is successful, however, the hydrothermal processes provide a great clean energy source.
Figure 4. [Geothermal Information, 2010]
Rainwater infiltrates bedrock, becomes superheated, and convects.
Producing Energy
The Geothermal Information Resources website reports four principal types of geothermal power plants are now used for the production of electricity. The first two are flash and dry steam power plants. Pumping techniques differ slightly, but both of these types of plants essentially use high-temperature hydrothermal systems to turn a turbine as hot water becomes exposed to low pressure and converts to steam. The next two are binary and binary/flash power plants that are used in moderate-temperature hydrothermal settings. A binary plant uses a secondary loop that usually contains a hydrocarbon fluid to be heated, vaporized, and pressurized to turn a turbine [Wagner]. A binary/flash plant combines this technique along with using the steam from superheated water to turn a second turbine. Binary geothermal power plants are ideal because they can operate in more areas with moderate-temperature waters. The production loop is not altogether complicated.
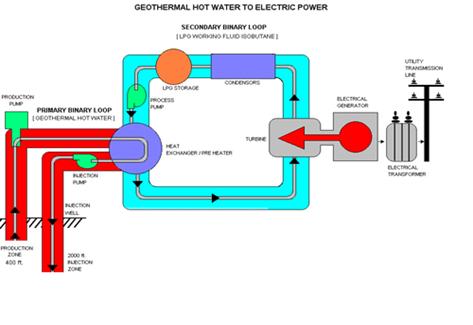
Figure 5. [Mammoth Pacific, 2003] Mammoth Pacific’s binary geothermal power plant design.
In example, Mammoth Pacific Geothermal Power Plant in Mammoth Lakes, California, has a total of three binary power plants in operation on site. First, a production well taps into the geothermal reservoir at approximately one hundred twenty meters below the surface, bringing up water that is around 150℃ in Mammoth Pacific’s case, says plant operations supervisor Andy Wagner. This water continues to be kept under pressure in the piping so it will not convert to steam. The hot water then enters a series of heat exchangers, transferring heat from the water to a hydrocarbon liquid, isobutane. Water leaves the heat exchangers at a much cooler temperature of around 70℃ and is pumped back into the ground through an injection well at a depth of six hundred meters.
Hydrocarbons like isobutane have a significantly lower boiling point than water and often exist in gas form even at room temperature. This makes hydrocarbons an ideal fluid choice to vaporize and pressurize in the binary loop. Heat from groundwater highly pressurizes the hydrocarbon vapor, and this vapor spins a turbine at up to 11,000 rpm [Wagner]. Isobutane is then sent through condensers and cooling systems to be “recycled” for the next round of heating and pressuring.
Through a series of coils, the turbine’s kinetic energy from the pressurized isobutane produces electrical power in a generator. The electricity can then be transmitted to a power grid for distribution. The plant runs a continuous binary loop of the geothermal water and hydrocarbon gas, making the most of its special geologic setting. Mammoth Pacific started operating binary plants in the 1980’s. The site’s three plants generate thirty-five to forty-five megawatts of power in total, enough to supply 40,000 homes, Wagner says.
Environmental Issues
Some are myth but some are fact: environmental impacts can still be seen as a result of this green geothermal energy production. Effects seem relatively minor now in comparison to other methods of producing electricity, but since this technology is so young, it is important to continue investigations of the repercussions geothermal energy production may cause.
Hydrocarbon Release
Even though plants keep hydrocarbons such as isobutane and isopentane in a closed loop and the fuel is not actually being burned, sometimes the gases will leak from storage tanks. Plants like Mammoth Pacific have to purchase isobutane two to three times per year (for a total of about 20,000 gallons for three plants) simply to replenish what is lost to the atmosphere [Wagner]. The hydrocarbons are denser than air and highly flammable, so it is crucial to monitor and help prevent leaks. If released in large quantities, the gases will sink and become concentrated near the surface of a topographic low, creating a major fire hazard especially in arid regions like the western United States.
Returning the Water
It seems sensible to process the water that geothermal plants bring to the surface for drinking water since pumping efforts have already been made. Another option would be to simply spill the water onto the ground surface and save energy by not having to inject the water back into the depths of the crust. This would prove to be extremely dangerous, however.
High temperature water that circulates deep underground around hot magmas has an increased ease of dissolving and transporting minerals. The water is considered as brine that is heavily laden with salts and metals in solution [Geothermal Information, 2010]. Bedrock’s composition changes as it interacts with hot water, and this can lead to dangerous levels of minerals or toxins in the circulating groundwater. This process is called hydrothermal alteration. Groundwater around geothermal plants can carry hazardous concentrations of elements like lead, cadmium, arsenic, and mercury [Wagner]. Sometimes the water is so harsh that is causes rusting or corrosion of power plants’ piping. Removing enough of these dissolved compounds from the pumped water would be a painstakingly lengthy and costly project, so it is not feasible to process this water for drinking. Spilling water onto the ground surface is also not feasible while it threatens animal and plant life, and it could ultimately contaminate other surface water resources.
In addition, if power plants do not return the water they pump out of the ground, subsidence may occur. Subsidence is sinking or lowering elevation of the ground due to reduced pressure below the surface [Sorey, 1995]. If a large water reservoir helps support and suspend the overlying landscape and suddenly too much of that water is pumped out of the reservoir, the ground above will subside or “deflate” as the elevation of such water table drops as well.
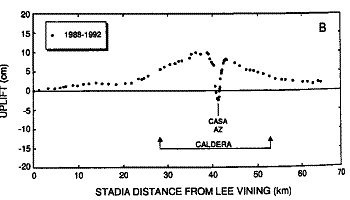
Figure 6. [Sorey, 1995] This graph shows evidence of subsidence (elevation changes with respect to Lee Vining, CA) in the Long Valley area after pumping for geothermal energy began in Casa Diablo, AZ.
Sorey [1995] cites effects on the ground elevation in the Long Valley, California, area where magmatic processes are creating a resurgent dome, but geothermal power plants’ operations have slowed the swelling of the surface. A resurgent dome in Long Valley had demonstrated an uplift of 0.5 meters from 1975-1988 and an uplift of 0.1 meters from 1988-1992. However, when compared to a benchmark outside of the resurgent dome, the area near Casa Diablo geothermal power plants had actually subsided 0.12 meters from 1988-1992 after they began tapping the reservoir for energy production [Sorey, 1995]. Even after returning the water, subsidence can still occur if circulation patterns within the reservoir are dramatically altered. For this reason, it is important for geothermal power plants to inject pumped water back into the ground in an effort to avoid subsidence and replenish the water for future use by the plant.
Sustainability
Geothermal energy is so sustainable because it uses energy from the earth that is already present as heat. Geothermal plants are quite efficient but still use about a third of their own produced energy to power other operations within the plant, like using cooling fans [Wagner]. However, the plants are smaller than fossil fuel-powered plants and attempt to conserve the aesthetic beauty of the land around them. “Visual pollution” is a very sensitive topic for the communities around power plants, and geothermal plants strive to minimize the effects that power production has on the landscape.
This type of energy production also gains much support from the environmentally-conscious community because it is a renewable technology. It is low-impact and geothermal reservoirs can be preserved for many years if managed correctly. Often binary plants can use “reclaimed” water to pump back into the ground, circulate and reheat, and drive power production [Duffield, 2003]. This sustains the life of the power plants even after a geothermal reservoir’s natural water supply has been depleted.
A Comparison to Coal Energy
As discussed earlier, geothermal power plants harvest already-present thermal energy. In contrast, coal-fired power plants, for example, must burn fossil fuels to create heat for steam production to turn turbines. Fossil fuel-burning plants must follow strict emissions regulations, but the fact still remains that these types of plants emit large quantities of particulates and nitrous oxide, carbon monoxide and dioxide, and sulfur dioxide gases that are harmful to the atmosphere [Miller]. It goes without saying that fossil fuel technologies are not “green,” and definitely not renewable. Advantageous aspects of geothermal energy are sustainability and essentially zero emissions.
Figure 7. [Duffield, 2003] This graph illustrates the difference in carbon emissions between fossil fuel-driven and geothermal-driven power plants. Burning of coal and natural gas emits as much as 900 kilograms of carbon dioxide per megawatt-hour, whereas geothermal-driven plants release only about 120 kg per megawatt-hour [Duffield, 2003]. No CO2 is emitted by binary geothermal plants.
The National Renewable Energy Laboratory, NREL, [2009] reported that in 2008, the United States geothermal energy production reached 15 billion kilowatt-hours, a mere 0.36% of the nation’s electrical production. Differences in magnitudes of power that geothermal plants versus fuel-burning plants can produce keep geothermal energy from being a large contributor to the power grid. One average sized coal-fired power plant will produce 1,000 megawatts of electricity compared to the mere 10-15 megawatts each plant at Mammoth Pacific generates [Miller and Wagner]. Since geothermal reservoirs are much less available and widespread for our use than fossil fuels, about seventy percent of the United States’ power supply comes from burning fossil fuels [NREL, 2009].
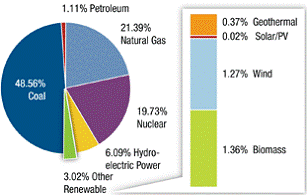
Figure 8. [NREL, 2009] This chart shows the 2008 contributors to electrical energy production in the United States. Geothermal energy plays a minor role to say the least.
Some areas like the Midwest are dependent on coal for affordable electricity as practically their only resource. Coal is widely accessible and effective for providing electricity to millions. Those unique places in the world like Mammoth Pacific are capable of reducing their carbon footprint though. Mammoth Pacific [2003] estimates saving 500,000 barrels of oil each year through their geothermal ventures. In Iceland, some 200,000 apartments make use of geothermal heat pumps and save around 170,000 tons of oil annually [Duffield, 2003].
Possible Improvements
Economically valuable geothermal reservoirs are often difficult to pinpoint everywhere in the world. This is due to the previously discussed limited predictability of the groundwater convectional patterns as well as a lack of more highly developed surveying technologies. The United States Geological Survey estimates that undiscovered geothermal energy sources could amount to a mean of 30,033 megawatts of electrical power [USGS Geothermal Resources Assessment, 2008]. Developing tools and methods to locate and utilize these possible undiscovered resources is a major improvement that can be made in the geothermal industry.
Efforts are also currently being made to research enhanced geothermal systems (EGS). Deeper crusts of the earth are hot across the globe, but not all such zones are made up of permeable bedrock. This means there is little to no water present, and this type of setting is referred to as “hot dry rock.” Geothermal Information [2010] says in order for this heat to be made available, engineers must find a way to make the hot dry rock permeable, pump water through it, and finally deliver the heated water to a geothermal plant. As mentioned earlier, some plants are starting to use reclaimed wastewater to pump back into their geothermal reservoirs to make more efficient use of the underlying hot rock.
Smaller Scale Geothermal Uses
One of the most economical heating and cooling resources comes from using geothermal energy. Households and businesses can use the constant subsurface temperature of the earth to regulate indoor climates efficiently. At as shallow as two to twenty meters, temperature stays constant at 15-20℃ [Geothermal Information, 2010]. (This is the same reason animals burrow in the earth for climate control.) Pipes conduct water in a closed earth loop running between the building and the ground. In the summer, the subsurface ground temperature is lower than that at the surface. Water that has absorbed heat from a building is cooled as it circulates through the loop, and this cool water is delivered back into the building to again absorb more heat and continue to cool the building. The process is the same for winter, except cold water circulating within a building is heated by the earth and delivers warmth back to the building.
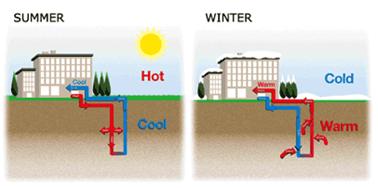
Figure 8. [Heat Pump Prices, 2010] This cartoon diagram
illustrates GHP’s heating and cooling abilities.
Use of geothermal heating pumps is so efficient and “green” because the only electrical energy required is used to simply pump water through the loop, not to actually produce hot or cold air. Georgia Air Contractors, Inc. [2010] reports that the earth loop technology is over four hundred percent efficient compared to that of about ninety-five percent efficiency of a fuel-burning system. In addition, the same system used to control air temperatures in the building can supply heat for hot tap water, making the method even more efficient. Geothermal heating pumps are usually more expensive to install than traditional heating and cooling systems, but their energy efficiency soon compensates for the difference and continues to save money by lowering monthly utility bills [Georgia Air, 2010].
In areas like Mammoth Lakes, California, where geothermal energy is a valuable resource and the climate provides a great amount of snowfall, geothermal energy can serve a very small-scale practical purpose. Coils with hot water running through them are often installed beneath sidewalks and driveways to aid in snow clearing [Duffield, 2003]. This saves time and labor as it uses heat that is simply passing through on its way to another greater destination, a very efficient operation.
Conclusion
There is little doubt that geothermal energy is a valuable resource. Geothermal energy also has a promising future as costs for natural gas and coal escalate, resources become diminished, and regulatory agencies continue to limit what fuel-burning companies can release to the atmosphere. However, geothermal energy may prove to be only more effective as heating and cooling systems since large-scale production is either out of the question or so underdeveloped that technologies to allow it will not be available for a long time. With that said, using heating and cooling systems driven by geothermal energy may significantly lower the per capita electricity consumption, thus essentially contributing more to (or saving) the power industry than is first evident. This is a field worthy of time and research investments to more fully understand it and to be able to reap the full benefits of this natural resource.
References
Duffield, W.A., and Sass, J.H., 2003, Geothermal Energy—Clean Power From the Earth’s
Heat: U.S. Geological Survey Circular 1249, 36 p.
"Geothermal." Georgia Air Contractors, Inc. Web. 08 June 2010.
<http://gaci.biz/geothermal>.
"Geothermal Information Resources." Geothermal Energy Association. Web. 03 June 2010. <http://www.geo-energy.org/>.
"Geothermal Technologies Program: U.S. Geothermal Resource Map." U.S. Department of
Energy, 13 Jan. 2006. Web. 05 June 2010. <http://www1.eere.energy.gov/geothermal/geomap.html>.
"How Does Geothermal Heating Work?" Heat Pump Prices, Geothermal Cooling and Heating Systems Reviews. Web. 08 June 2010. <http://www.heatpumpprices.org/how-does-geothermal-heating-work>.
Mammoth Pacific, LP. 2003. Web. 08 June 2010.
<http://www.mammothpacific.com/index.html>.
Miller, Kriss M., Operations Supervisor. Hoosier Energy Merom Generating Station. Personal interview. 5 June 2010.
National Renewable Energy Laboratory (U.S.). 2008 Geothermal Technologies Market Report. Rep. no. E 9.24:NREL 2864. Golden: National Renewable Energy Laboratory, 2009. U.S. Department of Energy, 10 Sept. 2009. Web. 3 June 2010. <http://www.nrel.gov/docs/fy09osti/46022.pdf>.
Nelson, Stephen A. "Mineral Stability." Mineral Stability and Phase Diagrams Introduction. Tulane University, 26 Nov. 2002. Web. 05 June 2010. <http://folk.ntnu.no/krill/ mineralogee/13.htm>.
Sorey, M. L., et al. "Effects of Geothermal Development on Deformation in the Long Valley Caldera, Eastern California, 1985-1994." Geophysical Research 100.B7 (1995). Print.
USGS Geothermal Resources Assessment Team. Assessment of Moderate- and High-Temperature Geothermal Resources of the United States. Issue brief no. 2008-3082. U.S. Geological Survey, 2008. Print.
Wagner, Andy. 2010 G190 Class Tour. Mammoth Pacific Geothermal Power Plant, Mammoth
Lakes. 17 May 2010.