Today, only 10 percent of the Earth is covered by glaciers compared to an astounding 30 percent during the Pleistocene glaciations, occurring from 1.8 million to 10,000 years B.P. [Sauchyn, 2003]. Today, glacial evidence is seen primarily in the landforms left behind, many of which may be seen within the United States in the Sierra Nevada mountain range. For the last 1.5 million years, glaciers have covered the Sierra Nevada, carving deep into the landscape forming canyons such as the beautiful Yosemite Valley in Yosemite National Park [Sauchyn, 2003]. Glacial deposits, mainly moraines made up of unconsolidated glacial till, are dated by glaciologists worldwide in order to determine each glacier’s response to climate variability as well as the rate of glacial flow [Erickson, 1996]. Today, the study of glaciers is increasingly important. The one-tenth of the Earth covered by ice will not outlast global warming; as each glacier melts, the ocean levels slowly rise. If the entirety of the Antarctic Ice Shelf melted, the sea level is expected to rise over 60 meters, flooding countless cities in coastal areas, most of which are heavy populated [Sauchyn, 2003]. For this reason, the work of glaciologists around the world is increasingly important, especially if the understanding of the effects of climate variability on a single glacier could save thousands, if not millions, of lives in the coming years.
2. Introduction:
The primary means of collecting glacial evidence is by direct observation of glacial landforms as well as glacial deposits such as erratic boulders or rock flour found within lake beds. Rock flour, for example is composed of clay sized particles of rocks and minerals that are primarily found suspended within glacial lakes [Sauchyn, 2003]. The rock flour within Owens Lake, near the eastern Sierra Nevada, for instance, is composed of quartz, feldspar, and biotite, the three main components of granodiorite, suggesting the rock flour found within the lake bed originated from the Sierra Nevada mountain range [Bischoff and Cummins, 2001]. Furthermore, rock flour, as well as glacial moraines, when paired with either cosmogenic nuclide dating or carbon dating, may be used to generate an accurate chronology of glacial advances in a particular area, simultaneously showing the effects of a variable climate [Phillips et al., 1996].
3. Observations:
Glaciers may be defined, simply, as a large mass of moving ice. There are many types of glaciers found worldwide, many of which are constrained by the surrounding topography. Glaciers of this type include cirque glaciers, valley glaciers, and piedmont glaciers; all of which are known as types of alpine, or mountain, glaciers. Cirque glaciers originate in high elevations near the peak of a mountain [Sugden and John, 1976]. These types of glaciers are responsible for the formation of the related landform, a cirque. As a cirque glacier advances near a pre-existing valley, created either by a previous period of glaciations or by running water, the glacier is then classified as a valley glacier [Sugden and John, 1976]. The bulk of alpine glaciations are due to multiple series of advance and retreat of valley glaciers. However, as the glacier reaches the base of the mountain, it is then classified as a piedmont glacier; the glacier flattens, often creating a fan shaped ice field, as it flows over flat ground at the edge of the valley [Sugden and John, 1976].
3.1 Effects of Glacial Erosion
Constrained by the topography, glaciers carve into the pre-existing landscape to form a new, glaciated topography staring landforms such as cirques, horns, arêtes, and glacial troughs. These types of landforms are formed strictly by glacial erosion through a process by which the bedrock is cut by the poorly sorted debris carried by the basal, or bottom, layers of a glacier [Sugden and John, 1976]. This process of erosion is better known as abrasion. Direct evidence of abrasion is seen in striations, or parallel grooves carved into the bedrock that distinctly show the direction of glacial flow [Erickson, 1996]. The same parallel grooves may be seen when a piece of wood is scored by sand paper, further proving the striations seen on the bedrock originated from glacial erosion. As rock fragments from the basal layers of the glacier continue to erode, a glacial polish is left behind in addition to striations; however, glacial polish often peels off, decreasing the amount of glacial evidence [Sugden and John, 1976]. Fine examples of glacial polish may be found on many of the volcanic domes seen in Yosemite National Park, including Lembert Dome, Fairview Dome, and Polly Dome [U.S. Geological Survey, 1987]. Abrasion also results in the production of rock flour, fine grained sediment consisting of mineral fragments [Erickson, 1996]. As the glacier melts, the rock flour produced is washed away and later deposited in a glacial lake, such as Owens Lake in the eastern Sierra Nevada. The rock flour remains suspended in the water, giving the water a blue-green coloring [Sugden and John, 1976]. Thus, the presence of rock flour in lake sediments suggests glacial activity in the surrounding topography and is often used by scientists to determine the extent of glaciation in the area as well as the number of advances made by a certain glacier [Bischoff and Cummins, 2001]. Though rock flour is used by many scientists in their research, the primary means of glacial evidence is gathered by direct observation of the landforms left behind.
Many glacial landforms may be found along the Sierra Nevada, from cirques to glacial troughs, all suggest an immense period of glaciations. After a cirque glacier is formed, it begins to form a cirque, an amphitheatre shaped cavity carved into the side of a mountain [Erickson, 1996]. Formed by abrasion, cirques are surrounded by steep walls, known as arêtes, and are sometimes filled with small glacial lakes [U.S. Geological Survey, 1987]. A pyramidal peak, known as a horn, is formed as three or more adjacent cirques are formed on the same peak [Erickson, 1996]. The better known glacial landform is a deep u-shaped valley called a glacial trough [Sugden and John, 1976].
Glacial landforms, including both a cirque and glacial trough (labeled U-shaped valley), are seen in the figure. Figure reproduced from Hayes [2009] (exact reproduction).
Formed by the abrasion of a valley glacier, the pre-existing valley is straightened, as seen in Yosemite Valley, as the viscous ice advances farther, removing all evidence of the previous valley and replacing it with a new set of depositional evidence of the current period of glaciations [Sugden and John, 1976].
3.2 Glacial Deposits:
As a glacier advances it slowly deposits debris from the basal layers of the glacier. There are two main types of glacial deposits: glacial till and stratified drift. Glacial till is directly deposited by a glacier and is neither sorted nor stratified [Erickson, 1996]. Furthermore, it is an unconsolidated collection of many different rock types, many of which are angular and feature striations [Sugden and John, 1976]. Glacial till is often classified by the layer from which the glacier deposited it; for example, basal till is deposited from the bottom of the glacier while ablation till is deposited from the top of the glacier as it begins to melt [Erickson, 1996]. Glacial till becomes both rounded and stratified as glacial melt water transports the previously deposited till downstream and deposits it once again in a standing body of water such as a glacial lake [Sugden and John, 1976]. Large linear deposits of glacial till, called moraines, are also classified by the position within the glacier from which they were deposited. Terminal moraines are deposited as a glacier retreats, marking the furthermost edge of the farthest advance; all subordinate terminal moraines marking the end of a secondary advance are called recessional moraines [Erickson, 1996]. Other moraines include lateral moraines, deposited from the sides of a glacier, and ground moraines, deposited from the basal layers of the glacier [Sugden and John, 1976]. The figure below shows the joining of two cirque glaciers to form a valley glacier as well as both a terminal moraine and a recessional moraine.
Figure reproduced from Cliffs Notes website [2009] (exact reproduction).
Many types of moraines, including both terminal and recessional moraines, may be observed in Lee Vining Canyon east of Yosemite National Park; however, many of the moraines deposited on the west side of the Sierra Nevada were eradicated by streams formed by glacial melt water, such as the Merced River [U. S. Geological Survey, 1987]. Similarly, glacial moraines may not be found in Yosemite Valley; although, many erratic boulders are scattered across the famous u-shaped valley and a small series of moraines may be found along Bridal Veil Meadow [Hayes, 2009]. Another type of glacial deposit, erratic boulders, is deposited randomly across the landscape, usually indicating the source of the glacier as well as the direction of glacial flow [Erickson, 1996]. Identifying features of the erratic boulders, such as the mineral or fossil content, assist in finding the source from which the glacier first plucked the deposit, the same means by which lithic fragments are used in studying volcanic rocks. All glacial deposits, big or small, are used to study glacial movement in depth using new techniques such as cosmogenic age dating.
3.3 Age Dating Techniques:
A glacial moraine unquestionably reveals valuable information concerning glacial advances, making moraines a useful tool when inferring glacial variability due to climate changes. Many scientists are interested in calculating the effects of climate change on a glacier’s advance; however, in order to accomplish such a feat, the glacier’s last advance must be dated using cosmogenic nuclide dating or one of the many other techniques used to date a moraine. Various techniques used to date a moraine deposition include surface exposure age dating, radiocarbon dating, cosmogenic nuclide dating, lichenometry, or u-series disequilibrium. A soil analysis may also be conducted; however, not all moraines contain enough soil in order to complete a successful analysis [Graham, 1997]. Other techniques used to date a glacial advance include pollen analysis and observing changes in tree-line altitudes which directly show climate variability [Sugden and John, 1976]. Lichenometry, surprisingly similar to dendrochronology, or tree ring dating, uses the age of lichens growing upon a moraine in order to date the moraine itself [Erickson, 1996]. Lichen, analogous to either an alga or fungi, grows outward radially, gradually increasing in size as it thrives on an exposed bedrock or terminal moraine [Graham, 1997]. The radial growth may be measured and compared to the age of the moraine on which the lichen grows, for the moraine is undoubtedly older then the flourishing lichen. Cosmogenic nuclide dating is used to determine how long a rock has been exposed to the atmosphere; however, the particular isotopes involved, Beryllium-10 and Aluminum-26, when exposed to silica and oxygen in quartz, are only able to provide scientists with a minimum age of exposure, meaning the bedrock tested may be hundreds of years older than the determined age [Sugden and John, 1976]. Information concerning the depositional age of a moraine or the denudation, or reduction of elevation due to erosion, of a moraine may be gained by taking multiple samples from a moraine at different depths [Schaller et al., 2009]. Once the samples have been collected, an analysis of cosmogenic nuclides may be completed in order to successfully determine the age of the surface exposure. Further tests could use several of these techniques in order to date the deposition of rock flour within a glacial lake, further assisting scientists with their studies of glaciers.
Further evidence is gathered using GIS, or the geographical information system, a computer program that includes mapping software that is useful for both geostatistical and geospatial analysis; although, primarily it is an information system that stores, analyzes, and presents geographic information with which the user may analyze and edit spatial data in order to better understand geological processes [Napieralski et al., 2007]. GIS is often used in order to create topographic profiles that may be used to study the rate of erosion of a glacial moraine or the process by which a glacial landform was created. Using the mapping software, GIS is able to arrange a collection of geomorphic features by altitude, ultimately allowing scientists to reconstruct a map of ancient climatic conditions, such as atmospheric circulation [Napieralski et al., 2007]. The innovative landform data may also be separated by common properties and observed in a new dataset or newly generated model in which the separated layers are able to represent variable themes, as is seen in the model below [Napieralski et al., 2007]. GIS, in short, is used in order to model relationships between sediments and the landforms surrounding them. Thus, the many applications of GIS, or the geographical information system, are a key component of a glaciologist’s work, facilitating their understanding of the glaciated landscapes they study.
Layers of glacial landforms, such as moraines and drumlins, have been separated in the figure above in order to show the order of deposition. Figure reproduced from Napieralski et al.[2007] (exact reproduction).
4. Interpretations:
Age dating of rock flour, rather than glacial moraines, is an important point in the work of many glaciologists who work in glacial valleys such as Yosemite Valley, in which little to no glacial evidence is present save the large glacial trough and a few erratic boulders along the countryside [U.S. Geological Survey, 1987]. James L. Bischoff and Kathleen Cummins generated a detailed timeline of glaciations in the eastern Sierra Nevada by studying the amount of rock flour present within Owens Lake [Bischoff and Cummins, 2001]. The lake, itself, is deep within Owens Valley, a u-shaped valley, and boasts a surprising geologic history, as the lake sediments suggest alternating periods of closed lake conditions and floods. The presence of
and Magnesium Silicate Hydroxides alone suggest periods of aridity as well as closed lake conditions; however, layers of sediment with little
suggest a period during which the lake overflowed, meaning the lake experienced an increase in water flow in response to a glacial retreat [Bischoff and Cummins, 2001]. Bischoff and Cummins determined that the rock flour established within Owens Lake is composed of quartz, feldspar, and biotite, the main components of the granodiorite found throughout the Sierra Nevada [2001]. They also found that the amount of rock flour established within Owens Lake is directly proportional to the intensity of glacial coverage in the area as well as the amount of glacial abrasion experienced by the landscape [Bischoff and Cummins, 2001]. Using cosmogenic nuclide dating, Bischoff and Cummins generated a detailed plot of glacial advances in the eastern Sierra Nevada, reporting that the most significant glacial advance occurred 78,000 years B.P. that continued to advance as Owens Lake overflowed from 73,000 to 65,000 years B.P. [2001]. The study also showed that during this period of glaciations, marking the beginning of the Wisconsin glaciations, the glaciers experienced low insolation, a measure of solar radiation, during summers and little variability in precipitation [Bischoff and Cummins, 2001]. The following graph was generated by comparing the amount of rock flour found in the lake sediments to a time scale representative of continuous periods of glaciations.
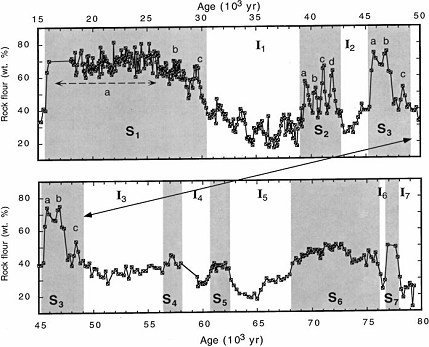
The shaded areas of the graph represent the seven maximums of glacial activity as shown by rock flour profusion. Figure reproduced from Bischoff and Cummins [2001] (exact reproduction).
The time scale in the graph above was constructed using data generated by cosmogenic nuclide dating of rock flour found in layers of lake sediment of Owens Lake near the eastern Sierra Nevada [Bischoff and Cummins, 2001]. The graph shows a total of seven glacial advances, represented by the seven maximum peaks of rock flour profusion separated by seven interstades, or periods of glacial retreat and warming [Bischoff and Cummins, 2001].
A previous study, conducted by Fred M. Phillips and his team of scientists, confirming similar results, ultimately showed that the size and extent of mountain glaciers are relatively close to equilibrium with the regional climate. Ice cores showed that during the last period of glaciations in the Sierra Nevada mountain range, the climate oscillated between periods of cooling, lasting around 500 to 2000 years each, and periods of warming due to large iceberg discharges [Phillips et al., 1996]. The team was unable to utilize carbon dating when sampling glacial moraines; however, cosmogenic nuclide dating was able to be applied due to a buildup of Chlorine-36 in glacial moraines found within four drainage areas of glacial melt water of the Sierra Nevada: Bishop Creek, Bloody Canyon, Chiatovich Creek, and Little McGee Creek, all shown in the figure below [Phillips et al., 1996].
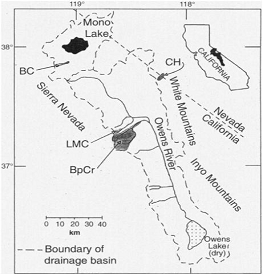
The figure shows the location of the Owens River drainage basin, in which are located the four areas from which moraines were dated using cosmogenic nuclide dating. The four drainage areas are as follows: CH-Chiatovich Creek, BC-Bloody Canyon, LMC-Little McGee Creek, and BpCr-Bishop Creek.
Figure reproduced from Phillips et al. [1996] (exact reproduction).
A second approach was then applied to the study using carbon dating on glacial proxies, primarily rock flour found within the sediment of Owens Lake, which showed a minor series of glacial advances occurring between 40 and 25 thousand years B.P. [Phillips et al., 1996]. The Owens Lake sediments also indicated a period of minimal glacial activity in the Sierra Nevada as the climate continued to change; however, the mountain range experienced a significantly large glacial advance, known as Tioga 2, as the climate became polar [Phillips et al., 1996]. The chronology generated by cosmogenic nuclide dating also showed that as the glaciers retreated, Owens Lake receded to near desiccation. The lake later reached its peak during the late Pleistocene glaciations as the glacial extent in the Sierra Nevada increased [Phillips et al., 1996].
5. Conclusion:
For the last 1.5 million years, glaciers have carved deep into the Sierra Nevada mountain range, leaving behind a magnificent collection of glacial landforms to be explored [Sauchyn, 2003]. From cirques to glacial deposits, each landform is a reminder of the power held by glaciers, moving fields of ice able to reconstruct ancient landscapes; however, as glaciologists continue to study the effects of climate variability on glaciers, a glacier’s response to global warming, an increasing threat, may be better understood. Every day, new applications, such as cosmogenic nuclide dating and U-series disequilibrium, are perfected, generating detailed chronologies of the glacial record worldwide and ultimately showing continued glacial advances during periods of both cooling and warming. Take into account the study completed by Fred Phillips and his team of scientists, in which climate variability effected not only the extent of glaciations within the Sierra Nevada mountain range but the state of Owens Lake as well, proving the growing importance of glacial studies, for as the sea level rises in response to an increased flow of glacial melt water, a better understanding of glacial processes could save thousands, if not millions, of lives in the coming years [Phillips et al., 1996].
Works Cited
Bischoff, J.L., and Cummins, K. (2001). Wisconsin Glaciation of the Sierra Nevada (79,000-15,000 yr B.P.) as Recorded by Rock Flour in Sediments of Owens Lake, California. Quaternary Research, 55 (1), 14-24.
CliffsNotes (2009). Glacial Deposits. Retrieved from http://www.cliffsnotes.com/WileyCDA/CliffsReviewTopic/Glacial-Deposits.topicArticleId-9605,articleId-9523.html
Erickson, J. (1996). Glacial Geology: How Ice Shapes the Land. New York, NY:
Facts On File, Inc.
Graham, D.J. (2007). The Neoglacial history fo Akshayuk Pass, Baffin Island, Canada, using Lichenometry and rock weathering techniques. Retrieved from http://www-staff.Iboro.ac.uk/~gydjg2/baffin/2_dating.htm#2.3
Hayes, G. (2009). Glaciation of the Sierra Nevada.
Retrieved from http://www.virtual.yosemite.cc.ca.us/ghayes/sierragla.htm
James, L. A. (2003). Glacial erosion and geomorphology in the northwest Sierra Nevada, CA. Geomorphology, 55, 283-303.
Napieralski, J., Harbor, J., and Li, Y. (2007). Glacial geomorphology and geographic Information systems. Earth Science Reviews, 85 (1-2), 1-22.
Phillips, F.M., Zreda, M.G., Benson, L.V., Plummer, M.A., Elmore, D., Sharma, P. (1996). Chronology for Fluctuations in Late Pleistocene Sierra Nevada Glaciers and Lakes. Science, 274, 749-751.
Sauchyn, D.J. (2003). Glacial Geomorphology. Retrieved from http:// www.uregina.ca/~sauchyn/geog221/
Schaller, M., Ehlers, T.A., Blum, J.D., and Kallenberg, M.A. (2009). Quantifying glacial moraine age, denudation,and soil mixing with cosmogenic nuclide depth profiles. Journal of Geophysical Research, 114. Doi: 10.1029/2007/JF000921
Sugden, D. E., and John, B. S. (1976). Glaciers and Landscape: A Geomorphological Approach. New York, NY: John Wiley & Sons.
U.S. Geological Survey. (1987). The Geological Story of Yosemite National Park
(Bulletin No. 1595). Washington, DC: U.S. Government Printing Office.