This paper examines the Bristlecone Pine (pinus longaeva), the longest-living, noncloning organism, and its importance to science. In order to better understand the region where the oldest bristlecones are found, the paper also discusses the geology and climate of the White Mountains . The White Mountains are located in the rainshadow of the Sierra Nevada in California . The dolomite outcrops, high alkaline content, and low precipitation (<12 cm/yr) are essential for the bristlecones. In addition the high altitude of 14,246 feet (4343m) is essential, since bristlecones grow best between 10,000 and 11,000 feet (3048 to 3354 m). Ed Schulman was the first to study bristlecones in depth. Bristlecones can live to be as old as 4,844-years-old, with several reaching ages of more than 3,000 to 4,000 years. Their ability to dieback onto the cambrium layer in addition to having highly resinous wood is essential to their longevity. Schulman's research, along with A. E. Douglas, led to the development of dendrochronology. Crossdating, the process of matching growth patterns among several tree series, is the foundation of dendrochronology. Bristlecones are especially important for dendrochronology since their chronological records go back about 11,000 years. These records have helped correct radiocarbon dating, allowing scientists to accurately date items to the correct year. Knowledge of bristlecones is essential for gaining a better understanding of the historical geologic and climactic state of regions.
INTRODUCTION
In the extreme conditions and high altitudes of the White- Inyo Mountains lives the world's longest-living, noncloning organism: the Bristlecone Pine (pinus longaeva) (Petrides). With the oldest recorded tree living to be 4,844 years old, it does not come as a surprise that these organisms appear to be more dead than alive (Hamburger). Bristlecone Pines are not only notable for their ability to survive for more than 40 centuries, but they also are great contributors to science. Their age and resinous wood makes them perfect subjects for studying growth rings. Climate histories have been recorded in the trees' growth rings, giving rise to the science of dendrochronology. In addition, growth rings of known age have enabled improved precision in the radiocarbon dating of ancient organic materials. (Petrides).
WHITE MOUNTAINS
The Ancient Bristlecone Pine Forest lies within Inyo National Forest , part of the White-Inyo Mountain range. According to Monroe, the White Mountains lay along the western edge of the Great Basin in east central California, extending for approximately 60 miles (100 km) narrowing to a point at the north, with elevations generally increasing south to north. According to James Monroe, “Fault-block or fault mountains are produced when normal (near vertical) faults fracture a section of continental crust. Vertical motion of the resulting blocks, sometimes accompanied by tilting, can then lead to high escarpments. These mountains are formed by the earth's crust being stretched and extended by tensional forces.” As is typical of the ranges in the Basin and Range Province , it is bounded by normal faults of large-magnitude slip (Nelson, et al.). White Mountain Peak is the highest point in the range, and the third highest summit in California , at 14,246 feet (4,343 m). This peak is actually an extinct volcano rising about 1,600 ft (500 meters) above the plateau surface ( Monroe ).
Geology
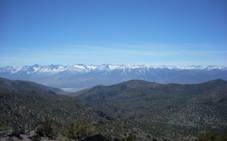
According to Brian Speer, “The White Mountains (pictured above: Photo Credit: Nathan Nahler), the Inyo Mountains, and associated ranges present one of the most important and best-known Late Proterozoic to Cambrian sections in the United States.” The mountains are home to unfossiliferous Precambrian rocks over 540 million years old from the Wyman Formation. Later Paleozoic rocks in addition to Precambrian and Cambrian can also be found to the south of the White-Inyo Range , in Death Valley and throughout the Mojave Desert (Speers). The central section of the range exposes the best stratigraphic sections of the uppermost Precambrian to middle Paleozoic strata (Nelson, et al.). Dating features within the range is difficult because of the large gap between the deformed and undeformed strata, with Mesozoic intrusive bodies truncating many of the strata. The major folding and thrust faulting that is present throughout the range generally happened before 180 Ma, and thus is pre-intrusive, or pre-Nevadan (Nelson et al.). These pre-intrusive structures are disrupted by plutons that have metamorphosed the sedimentary strata to slate, schist, and quartzite. Some of the sedimentary layers have been strongly deformed and stretched from the plutons. These plutons range in age from 180-75 Ma (Nelson, et al).
The White Mountains are composed of quartzitic sandstone and granite bedrock (Miller). Extensive outcrops of dolomite, a type of limestone, can be found throughout the range. According to Miller, dolomite is “a very ancient rock first laid down under water 500 million years ago, then slowly uplifted through time.” Though dolomite is very low in nutrients, it is able to hold a higher amount of moisture than the surrounding sandstone. Dolomite easily reflects sunlight, thus allowing for cooler root (Millar). The dolomite is surrounded by granite and sandstone formations that support Limber Pines and sagebrush. The bristlecones are mostly restricted to the areas of alkaline dolomite (Hughes and Graumlich).
Climate
Rising to a height of 14,246 feet (4343m), the White Mountains receive an annual precipitation of less than 12 inches (30cm), according to Miller. The large variation in elevations, from 4,000–5,000 ft (1,200–1,500 m) at the base to 14,246 ft (4,343 m) at the summit, results in intense changes temperature and precipitation within short horizontal distances (Powell and Klieforth). Although heavy precipitation from Pacific storms falls on the upper western slope of the Sierra (up to 80 in 200 cm of water annually), the White Mountains are in the rain shadow of the Sierra, thus receiving much less precipitation, (Powell and Klieforth). During the summer, the amount of precipital moisture found in the air is about half a millimeter (Miller). Unlike the Sierra Nevada, the White Mountains receive a majority of their precipitation in the spring, due to closed cyclones over the region (Powell and Klieforth). Average temperatures range from 70 F (21C) to (37F (3C) at the base and from 36 F (2 C) to -26F (-32C) in the alpine zone (Miller). However, a strong northerly fetch of air may bring Arctic air from Alaska or the Yukon . Through this happens on infrequent occasions, usually separated by several years (e.g., January 1937, January 1949, December 1972, February 1989, and December 1990), the result is record cold temperatures (Powell and Klieforth). In addition, the White-Inyo Range is subjected to strong winds and frequently occurring thunder and lightning storms. Extreme conditions have eroded a large amount of the soil on the range. Whatever soil does remain is of very poor quality, especially in the alpine zone (Miller). Since most plant life cannot live in this barren soil, the area is essentially competition-free. It is because of these extreme conditions that the White Mountains are home to the biological wonder that is the Bristlecone Pines.
HISTORY
While Bristlecone Pines have been living since two millennia before Christ, their longevity was a secret until the 1950s. According to Miller, starting in 1932, Edmund Schulman worked as an assistant to A. E. Douglass at the Laboratory of Tree-Ring Research, University of Arizona . Climate records in the western United States at that time were no more than a few centuries old. Originally, Schulman focused his work with climate conditions on pinon and Douglas-firs in the lower forest zones. Schulman then realized trees in the upper forest zones that were subjected to much more stressful conditions would have more sensitive records of drought in their growth rings. Schulman refocused his work in upper forest zones. After finding a 600-year-old Douglas fir, 800-year-old bristlecone, and 975-year-old pinon, among others, Schulman began reconstructing a climatic history of the area. By observing growth rings, Schulman inferred that the area underwent 200 year cycles of flooding and droughts, starting in A.D.1215 (Miller).
Schulman and Bristlecones
According to Miller, Schulman continued to pursue his study of dendrochronology through the 1950s. It was in 1953 that, along with his colleague Frits W. Went of Cal Tech, he found a limber pine with 1700 growth rings in Sun Valley , Idaho (Miller). Schulman decided to head back west after hearing a rumor that the White Mountains were home to even older trees. A local ranger showed him “Patriarch”: a multiple-stemmed bristlecone that was 36 feet in circumference (Miller). After taking core samples, Schulman learned the tree was 1500 years. While it was not the oldest tree he had found, it did imply that bristlecones were more important than previously thought. Due to the harsh conditions they lived in, they proved to be more useful than Limber Pines for deducing drought records. Schulman then spent the next two years (1954-1955) researching locations of Bristlecone Pines. The trees could be found from California to Colorado ; however, Schulman learned that the oldest trees lived at elevations of 10,000 to 11,000 feet (3048 to 3354 m). What was even more remarkable is that the oldest trees seemed to be the ones subjected to the harshest conditions. Schulman discovered several trees more than 3,000 and 4,000 years old; all except one were found in the White-Inyo Range (Miller).
In 1957, Schulman discovered “Methusalah”, the oldest known living tree (Miller). Today, “Methusalah” is 4,774 years old. A year later, Schulman passed away, right before his data was reported in National Geographic. The magazine brought global attention to the White Mountains . Within the same year, the U.S. Forest Service established the Ancient Bristlecone Pine Forest. The 28,000 acre park also includes Schulman Memorial Grove. In 1970, biologist D.K. Bailey found that the westernmost bristlecones had strikingly different cones and needles. He proved the differences were significant enough to warrant a new species, Pinus longaeva. The bristlecones to the east are called Pinus aristata.
Though “Methusalah” is currently the oldest living bristlecone, it was not the oldest to have been found. In 1964, a graduate student researching dendrochronology in the Great Basin found a tree, “Prometheus”, that he wanted to date. To do so, he gained permission to cut it down. After counting the rings, he learned it was about 4,900 years old (Hamburger).
PHYSICAL CHARACTERISTICS
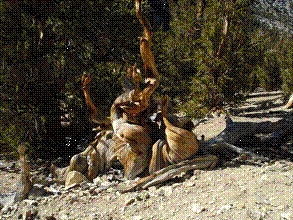
Going by strictly physical descriptions, bristlecones aren't particularly striking. They do not grow very tall; the tallest reach a rare height of 60 feet. On average they are between 15 and 40 feet (as shown by the picture above. Photo credit: Liz Bell). Their needles grow in clusters of 5, dark green, and only 1-1 ½ inches long. Their cones are cylindrical, thin-scaled, 3- 3 ½ in length, with rounded bases (Perides). The species is extremely slow growing; each year the tree increases its girth at most by 1/100 th of an inch (Miller). After 700 years, it can be just 3 feet tall. Lifeless trunks may stand for 1,000 years after death (Perides). Bristlecones only grow during three warm summer months. Often times, this period is limited to about six weeks with approximately 10 inches (25.4 cm) of precipitation. The trees that are anchored to more moist slopes grow more in size and girth, but often die off at earlier ages due to their less dense wood. The trees growing in the soil with least moisture and nutrients and most alkaline tend to live longer and grow slower. (Speers).
SURVIVAL STRATEGIES
Obviously bristlecones have developed survival strategies that are imperative to living so long. One key characteristic is that bristlecones are able to retain their needles for up to 40 years (Millar). Because of this, even if they have a decade or two of drought, they do not have to worry about producing new needles. These long living needles allow trees to sustain themselves over years of stress, since they provide stable photosynthetic capacity. In addition to long living needles, bristlecones are able to endure dieback (as shown in the picture to the left. Photo Credit: Liz Bell), during which time the tree can reduce its tissue if it is unable to support it. Bristlecones can survive for thousands of years with just one side growing (Millar). For example, “Pine Alpha”, the first tree found
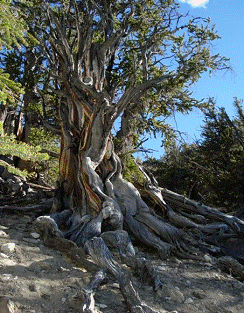
to be more than 4,000 years old, is nearly four feet in diameter, but only has a 10 inch strip of living bark. This ability allows a tree to still survive even if it is subjected to fire, lightning, drought, or intense storms (Miller). The living wood of the tree is highly resinous. This characteristic protects it from invading bacteria, fungi, and insects (Miller). The dry air surrounding the tree helps preserve it from rotting. Apart from these survival skills, the bristlecone's ability to live in such harsh climates helps it live longer as well, since it is not subject to any competition (Millar). Since the root system is able to spread out as much as needed, the trees are lavishly spaced. The trees are also spaced out because of water and nutrient restraints. Thus, when a fire catches, it is not able to easily jump from tree to tree (Millar). All of these characteristics combined allow the trees to live so long.
Limber Competition
As stated earlier, bristlecones are not used to competition since they live in such extreme conditions. However according to Millar, the limber pine, which is also found in the White Mountains (though normally at lower altitudes), has begun to colonize up and down the range. Limbers are appearing to become more adaptable to climate change. Because of this, they are colonizing at the expense of the bristlecones. Since the bristlecones are not used to this competition, they are unaware of how to respond to the new threat. However, this increasing colonization of the limbers is not new. According to Miller, the dominant tree goes in phases over the last 10,000. This could just be a new phase where limbers are more prominent.
Dendrochronology
Dendrochronology is the science that dates past events, such as climate change, through study of tree ring growth. By studying dated growth rings, scientists can analyze the patterns and environmental effects that caused the rings to grow the way they did. Though it is popularly used to infer climate change, it is also used to learn more about ecology, geomorphology, glaciology, hydrology, pyrochronology, archaeology, and entomology (Grissino-Mayer).
According to Miller, the science was developed in the early 1900s when A. E. Douglas realized that the width of rings differed based on precipitation. That is, wet years yield wide rings whereas dry years result in narrow rings. After further study, the science became more involved and scientists began to realize how much information growth rings could provide. According to Millar, an individual ring is a layer of cells produced by a tree in one year. Within the layer are two groups of cells: earlywood, the result of thin-walled cells from early in the season and latewood, thicker-walled cells produced later (Millar). Together, they form one annual ring (Grissino-Mayer). The contrast between one year's latewood and following year's earlywood is enough to establish a ring, thus making counting possible (Miller). The width of the ring is affected by precipitation, CO2, temperature, sunlight, soil nutrients, nitrogen, and genetic composition. However, scientists have to beware of false rings that are caused when a tree starts to shut down for the winter, but then the weather gets nice again and the tree begins to produce new cells, even though it is not a new year (Millar).
Uniformitarian Principle
Knowing what environmental conditions caused certain rings to form provides scientists with a plethora of information. According to Grissino-Mayer, the uniformitarian principle “states that physical and biological processes that link current environmental processes with current patterns of tree growth must have been in operation in the past.” Because of this, scientists gain a great deal of knowledge about an area's ecological history. In addition, the growth ring history can help scientists predict what an area will face in the future. For example, Schulman, as noted earlier, recognized 200 year cycles in droughts. It is feasible to assume, based on past precipitation records, that this cycle will continue. Often, we can use growth rings as a proxy for recorded precipitation prior to the historical record (Millar).
Limiting Factors
A tree relies on several inputs in order to grow. When one or more of these inputs is altered or not present, the tree will not grow as well (Grissino-Mayer). The factors that can impact growth this way are called limiting factors. A tree can only grow as fast as its most limiting factor allows. For instance, if a tree is in an area that receives very little rain fall, precipitation would be its most limiting factor. The growth of the tree would be completely reliant on the amount of precipitation it received. Different regions are controlled by different limiting factors. In some areas, latitude, temperature, nutrients, or environmental stressors could be the limiting factor. The bristlecone pine can survive a lot: lack of rainfall, lack of nutrients, etc. However, it is greatly affected by altitude (Millar). According to Miller, it lives best at 10,000 to 11,000 feet (3048 to 3354 m). Since the bristlecones live at the tree line, cold especially is the limiting climatic factor. In such a cold climate, it is hard for photosynthesis to take place since heat, not just light, is a necessary component (Valmore). The growth rings will be affected if it begins receiving too much or too little water, or if another organism begins to compete with it (Millar). This is rare though, since bristlecones live in very harsh regions where most other organisms cannot survive.
Aggregate Tree Growth
According to Grissino-Mayer, when looking at how a tree grows, scientists break it up into the environmental factors that impacted its growth over the years. The equation below shows how tree ring growth is compiled.

1. the age related growth trend (A) due to normal physiological aging processes
2. the climate (C) that occurred during that year
3. the occurrence of disturbance factors within the forest stand (for example, a blow down of trees), indicated by D1,
4. the occurrence of disturbance factors from outside the forest stand (for example, an insect outbreak that defoliates the trees, causing growth reduction), indicated by D2, and
5. random (error) processes (E) not accounted for by these other processes.
(The Greek letter in front of D1 and D2 indicates either a "0" for absence or "1" for presence of the disturbance signal.)
(Both this graphic and caption are direct examples from Grissino-Mayer)
When scientists need to target a specific environmental signal, they maximize its signal by minizimizing the others. For instance, Grissino-Mayer gives the example that “to maximize the climate signal, the age related trend should be removed, and trees and sites selected to minimize the possibility of internal and external ecological processes affecting tree growth.”
Other Key Principles
The principle of ecological amplitude states that the range of habitats in which a species may grow and reproduce over is called its ecological amplitude. Most useful trees for dendrochronology are found near the boundaries of their natural habitat (Gressino-Mayer). This is why bristlecones are so important, since they live at extremes (Millar). The principle of site selection states that when choosing sites for dendrochronological research, scientist should focus on the environmental variable being examined (Grissino-Mayer). Thus, if a scientist is focusing on drought conditions would chose areas that receive limited amounts of precipitation.
Crossdating
Crossdating is the process of matching ring patterns among several tree-ring series in order to identify the exact year a tree ring was formed (Grissino-Mayer). This is the basis of dendrochronology that allows scientists to set up actual chronologies. When building chronologies, dendrochronologists use averages of multiple trees, thus eliminating individual variations. This process is called replication (Friedrich). By applying crossdating and replication principles to bristlecones, scientists have compiled a roughly 8,702year-long chronology (Stiles). This does not include the unconnected floating 3,000 year old chronology, whose beginning and end dates are not known (Friedrich). If scientists are able to link the two, the resulting chronology would extend back to the last Ice Age (Stiles). The bristlecone's highly resinous and disease/insect resistant wood is key to preserving trees even after they have been dead for thousands of years (Miller). Because of this, scientists are able to compile floating records and extend current chronologies.
Importance of Bristlecones
Bristlcones are particularly good climate signals because they live along the tree line, at the extreme edge of the conditions (Millar). Also, because the Methusalah Walk region is highly moisture-stressed, the crossdating between bristlecones is very clear. Also, replication is particularly strong, seeing as there are at least 10 samples for every year since 6,000 B.C. through AD 1995 (Hughes, et al). In addition, the unusually long lifespan of bristlecones helps when looking for erosion and soil uplift over the last 5,000 years just by looking at one tree. Scientists can also easily see the shift of the tree line over a long period of time just by observing this one species (Millar).
Radiocarbon dating
Another impact that bristlcones and dendrochronology have had on the scientific world is helping correct radiocarbon dating. Radiocardbon dating relies on radioisotope carbon-14 to determine the age of carbonaceous materials up to between 50,000 and 60,000 years old (Plastino, et al.). According to W. Plastino, “Uncalibrated radiocarbon ages are reported in Before Present (BP)” terms, with AD 1950 being “present”. Radiocarbon dating was developed by Willard Libby, a scientist who worked on developing the nuclear bomb in World War II. Since every living thing on earth has carbon in it, it is perfect for dating (Petchy). However, radiocarbon is not exactly accurate, which is where dendrochronology comes in. Since tree-rings are exact and scientists are able to crossdate and build chronologies, radiocarbon daters can compare these chronologies with the information they have found (Petchy). Similarly, if dendrochronologists find a floating chronology, they can date it using radiocarbon and get an idea of the time frame. They can then cross date to find the exact age. Thus, dendrochronology and radiocarbon dating form a nice check and balance system that is helpful for archaeologists, climatologists, and many other scientists. Bristlecones are accessible specimens of once-living, accurately dated material that can be used to check radiocarbon dating (Renfre). Since the bristlecone chronology goes back more than 10,000 years, it is especially helpful for this purpose (Hughes and Graumlich).
Conclusion
The Bristlecone Pine is a unique organism that has found the key to longevity. Apart from providing interesting information on survival and longevity, bristlecones also play a crucial role in dendrochonrology. Their impressive age, ability to stay standing for 1,000 after they've died, and highly resilient wood makes them perfect subjects for dendrochronology. Dendrochronoligists have comprised chronology based off of bristlecones that dates back nearly 11,000 years. This chronology in addition to other findings have helped correct radiocarbon dating.
SOURCES
Friedrich, Remmele M, Kromer B, Hofmann J, Spurk M, Kaiser KF, Orcel C, Küppers M (2004). "The 12,460-year Hohenheim oak and pine tree-ring chronology from central Europe - A unique annual record for radiocarbon calibration and paleo-environment reconstructions". Radiocarbon 46: 1111–1122.
Hamburger, M.W. and Connie Millar, 2008, Field notebook for “Volcanes of the Eastern Sierra Nevada,” unpublished field guide, Indiana University .
Hughes, Malcom K., Funkhouser Gary, Ni Fenbiag. “The Ancient Bristlecone Pines of Methusalah Walk , California , as a Natural Archive of Past Environment.” Science Highlights. PAGES. Vol 10. N 1. April 2002.
Hughes, Malcom K and Lisa J. Graumlich. “Multimillennial dendroclimatic studies from the western United States ,” Laboratory of Tree-Ring Research, University of Arizona .
Millar, Connie. Interviews via web, May 28-June 5, 2008.
Miller, Leonard. “The Ancient Bristlecone Pine”. www.sonic.net/bristlecone/intro.html .
Monroe, James S., and Reed Wicander. The Changing Earth: Exploring Geology and Evolution. 2nd ed. Belmont : Wadsworth Publishing Company, 1997.
Nelson, Clemens; Clarence A. Hall, Jr., and W. G. Ernst. Geologic History of the White-Inyo Range. Natural History of the White Inyo Range. http://www.escholarship.org/.
Petchy, Dr. Fiona. “Radiocarbon WEBinfo site,” www.c14dating.com/k12.html.
Radiocarbon Dating Laboratory, University of Waikato, New Zealand.
Petrides, George A; Olivia Petrides. Western Trees. Peterson Field Guides. Houghton Mifflin company. New york . 1998. First addition.
Plastino, W.; Kaihola, L.; Bartolomei, P.; Bella, F. (2001). "Cosmic Background Reduction In The Radiocarbon Measurement By Scintillation Spectrometry At The Underground Laboratory Of Gran Sasso". Radiocarbon 43 (2A): 157–161.
Powell, Douglas R and Harold E. Klieforth. Weather and Climate.
Natural History of the White Inyo Range. http://www.escholarship.org/editions/ view?docId=ft3t1nb2pn&chunk.id=d0e306&toc.depth=1&toc.id=d0e306&brand=eschol
Renfre Colin, Bahn Paul. Archaeology: Theories, Methods and Practice, Fourth Edition, 144-145.
Speer, Brian R. “Cambrian.” 22 November, 1994. Accessed 8 June, 2008. http://www.ucmp.berkeley.edu/cambrian/whites.html
Stiles, Lori. “Climate Quest: Volunteers search among ancient trees,” Report on Research, Laboratory of Tree-Ring Research.
Valmore C. LaMarche, Jr., "Environment in Relation to Age of Bristlecone Pines," Ecology, Vol. 50, no. 1 (1969), map, p. 57.
|