Introduction
Glaciers are more than they probably appear to be at first glance. Certainly many modern alpine glaciers would seem nothing more than large, unobtrusive patches of snow to the casual observer. One would not necessarily guess that they are the products of tens, hundreds, or thousands of years' worth of snow, which has been compacted under its own weight to nearly the density of water [Hill, 2006], or that members of their kind can carve razor edges out of rounded peaks and grind down the tops of hills. The idea of a time in history when much of the Earth was cold and covered with ice and snow is now well accepted, but this has not long been the case. It was only in the mid-nineteenth century that this idea of a wide-spread Ice Age first came to be. Since then, scientists have taken great interest—first in proving or disproving it, and more recently in chronicling Ice Age events through their deposits. By now, scientists have managed to construct a fairly detailed (if not indisputably correct) chronology of glacial events during the last 1,500,000 years or so, and for many regions, they also have some notion of one or more earlier glaciations. I attempt here to provide an overview of past and current thoughts on how and why glaciers are born, the landforms they produce, and what they have accomplished in the Sierra Nevada.
Old Ideas, New Ideas
Floods and icebergs
Although widespread glaciation is a relatively new idea, people have noticed and wondered about its traces for a long time. Europeans, hundreds of years ago, saw rock debris that appeared to have come from elsewhere and concluded that it must either have been swept in by raging flood waters or have floated by way of icebergs [Tarbuck and Lutgens, 1982]. Their theory is the reason why today, despite what we now know, we still use glacial drift as a general term for all sediment brought to its place by glaciers [Tarbuck and Lutgens, 1982].
They move!
It was in the late eighteenth and early nineteenth centuries in the Swiss and French Alps that farmers and herders saw that the angular boulders in their valleys matched rocks farther uphill and voiced their opinions that this was due to the valleys' glaciers having once extended farther down [Tarbuck and Lutgens, 1982]. This implied that glaciers moved—a concept that met with significant opposition. As a test, stakes were set in a straight line across a glacier. Despite the fact that it was retreating, all the stakes moved downhill, with the ones in the middle advancing faster than the ones at the edges. It was true, then, that the ice moved, and not at a uniform rate [Tarbuck and Lutgens, 1982].
A Widespread phenomenon
The idea of an Ice Age began to take shape in Europe in 1837 when Louis Agassiz wrote about evidence such as this, indicating that glaciers in the Alps had, at one time, been more extensive. Assuming that the alpine glaciers had reached farther down their valleys in the past, perhaps even onto the plains, Agassiz generalized and suggested a “great ice period” during which much of Europe and Asia had been glaciated [Guyton, 1998]. The concept had been set forth before and dismissed, but as Agassiz was well-known and respected, his assertion prompted scientists, skeptical though they were, to take to the field in search of evidence one way or the other. Many were still unconvinced when, twenty-six years later, the path of a California glacier was discovered [Guyton, 1998].
Once it was established that an Ice Age had taken place, the assumption was that there had been only one. Eventually evidence of others was found, including tentative traces of one in California [Guyton, 1998]. It is now accepted that Ice Ages are regular occurrences, but we still tend to use the term in the singular, referring to the most recent one, also known as the Pleistocene Ice Age. The term comes from the Pleistocene Epoch (1,800,000-10,000 years ago), which immediately preceded our present Holocene , and during which the bulk of recent glaciation took place.
Climate Change
Celestial cycles
What seems to affect climate the most over time, and therefore glacial formation, is a triad of astronomical cycles discovered by Milutin Milankovitch in the 1920s [Botkin and Keller, 2007]. The first of these is the variation in Earth's orbit, which is a cycle of about 100,000 years, and with which the major glacier and interglacial periods correlate rather well. The other two cycles are of 40,000 and 20,000 years respectively and represent the tilt of Earth's axis relative to the plane of its orbit and the degree to which its axis wobbles [Botkin and Keller, 2007]. These three reinforce each other at times, causing extreme warming or cooling, and at other times counteract one another to produce moderate temperatures [Guyton, 1998]. The timing of these cycles can be calculated, and the glacial record reflects our calculations to a great extent, though not perfectly, indicating the influence of some additional factor [Guyton, 1998]. One possibility is variation in the sun's activity. High ratios of isotopes from the sun's rays, which have been found in ice core samples, suggest that the amount of solar energy reaching the Earth during the medieval warm period (about 1100 to 1300 A.D.) was relatively high. Lower ratios from around the fourteenth century suggest that less solar energy was reaching Earth when the Little Ice Age began, about 700 years ago [Botkin and Keller, 2007]. Still, according to Botkin and Keller [2007], these astronomical happenings by themselves could not produce as wide a range of temperatures as the Earth has experienced, so there must be yet another factor.
Geological goings-on
Plate tectonics seem to account for most of the rest of Earth's climatic variability through several different processes. Tarbuck and Lutgens [1982] state that, along with suitable astronomical conditions, the placement of landmasses in high latitudes is required in order for widespread glaciation to occur. This is because glaciers can only form where 1) there is land and 2) it is cool enough for some snow to remain year round. Some scientists think that, as a rule, ice ages begin when land moves into polar regions [Tarbeck and Lutgens, 1982]. Also controlled by shifting plates are the uplift of mountains, which changes air current, and the reconfiguration of ocean basins, which changes water current [Guyton, 1998]. Both types of current exert strong local influence over temperature and precipitation.
In contrast to these more gradual sources of geologically prompted climatic change, large volcanic explosions can cause more sudden and conspicuous cooling [Botkin and Keller, 2007; Guyton, 1998]. The Long Valley eruption of 760,000 years ago was certainly large enough to have this effect, and, in 1991 and 1992, global warming was essentially counterbalanced by cooling resulting from the much smaller, but still quite sizeable, eruption of Mount Pinatubo in the Philippines [Botkin and Keller, 2007]. Volcanoes can have this effect because of the aerosols they release, including sulfur dioxide. Aerosols are particles so small that they are affected more by collisions with air molecules than by gravity, which means they can stay suspended in the air for long periods of time. The quantities of aerosols released into the atmosphere by an eruption such as that of Mount Pinatubo act as a sort of “dust veil” which reflects light rather than allowing it to reach the Earth [Botkin and Keller, 2007]. In addition, they provide a surface on which for clouds to form. The clouds, in turn, reflect more solar energy [Botkin and Keller, 2007]. A volcanic eruption alone would most likely not cause severe enough cooling for glaciers to form, but if astronomical factors also dictate some degree of cooling, it may be enough to tip the scale [Botkin and Keller, 2007]. Once in place, glaciers reflect the sun's rays to such a degree that they tend to perpetuate themselves [Guyton, 1998].
Anatomy of a Glacier
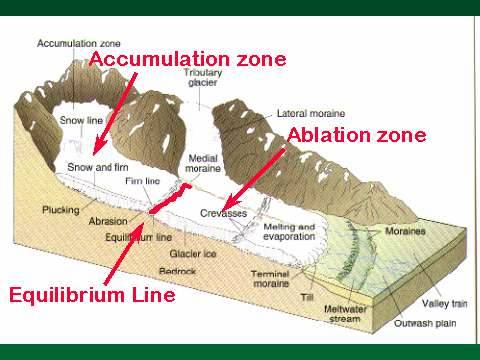
Parts of a glacier. Figure reproduced from http://www.geocities.com/geoamanda.
Glaciers form when snowfall exceeds snowmelt over a number of years. The area in which snow is being slowly transformed into ice is known as the zone of accumulation , and the area where melting occurs is the zone of ablation . The line between the two parts is called the firn line , as snow in the process of becoming glacial ice is called firn (“old snow” in German) [Guyton, 1998]. A glacier in equilibrium—neither advancing nor retreating—will be one third zone of ablation and two thirds zone of accumulation [Guyton, 1998]. All glaciers, even when “retreating,” will flow forward, downhill. This is because, by definition, they are massive enough that the stress of gravity is greater than the force holding together the particles of water [Guyton, 1998]. Such internal deformation begins to occur at a thickness of about 120 feet, which is the reason any fissures, or crevasses , in the ice are unlikely to exceed this depth. Glaciers also move by sliding over rock surfaces with the aid of meltwater in between. This is how they are able to abrade when carrying debris.
Types of Glaciers
Glaciers can be categorized in two main ways—by temperature and by location. Polar or cold glaciers inhabit high latitudes or very high altitudes, and their ice is colder than its melting point all year [Guyton, 1998]. Temperate or warm glaciers generally occur at high altitudes in low or moderate latitudes. Usually, most of their ice is at its melting point most or all of the year. There is more water in and under them, they release more water in the summer, and they are more erosive than cold glaciers [Guyton, 1998]. All the glaciers in California today are temperate, and judging from the latitude and sediment records, those that were there during the Ice Age probably were as well [Guyton, 1998]. All California glaciers are also confined or mountain glaciers . These, also, are of two main types. Cirque glaciers form in irregularities on mountain slopes [Guyton, 1998] and are confined to the cirques they create, which are amphitheater-shapes with steep walls [Hill, 2006].
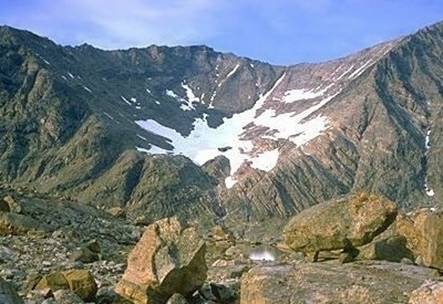
A cirque. Figure courtesy Natural Resources Canada, reproduced from geology.about.com/library/bl/images/blcirque.htm .
Valley glaciers start as cirque glaciers that then advance to occupy stream valleys. Unconfined glaciers are not contained by topographical features but are free to spread out in all directions [Guyton, 1998]. These are further classified by size. Ice caps , officially, are less than 50,000 square kilometers in area and may form either in mountains or on plains. When they encounter divides, they simply override them [Guyton, 1998]. Ice sheets , or continental glaciers , are the larger versions. They can be millions of square miles in area and up to two miles thick. Seemingly, the ice sheets of North America did not reach California during the Ice Age, but they did cover vast expanses of land farther north and east [Guyton, 1998].
Glacial Landforms
Landforms shaped by glaciation may be either erosional or depositional. Cirques, as mentioned earlier, are some of the most commonly found erosional glacial forms in the Sierra Nevada and often contain little lakes called tarns once their glaciers have gone. U-shaped valleys are also abundant. These are simply valleys that were formerly V-shaped, as cut by their streams, but have been occupied and eroded by valley glaciers. They have been rounded out to accommodate the glaciers' breadth and straightened into paths of less resistance [Hill, 2006]. Hanging valleys tend to form where tributary valley glaciers meet larger, deeper trunks and are frequently graced with modern waterfalls [Hill, 2006]. Yosemite National Park is home to some classic U-shaped and hanging valleys such as Yosemite Valley itself and the high troughs from which Yosemite and Bridal Veil Falls tumble. An arête (“rib” in French) may be formed by back-to-back cirques or by parallel valley glaciers. In either case, by basal sapping, which involves water from the glacier penetrating cracks in rock, freezing there, and acting as a lever to pry out boulders, glaciers progressively eat away at the separating rock until only a sharp ridge is left [Guyton, 1998].
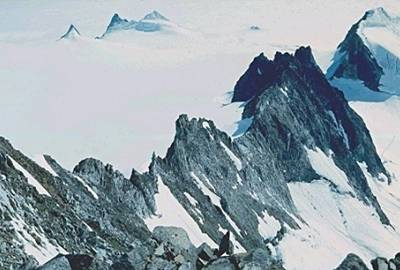
An arête still surrounded by glaciers. Figure reproduced from http://geology.about.com/library/bl/images/blarete.htm .
When three or more cirques form adjacently, they may leave a steep, pyramid-shaped peak in between called a horn [Hill, 2006]. Mount Whitney, the highest peak in the Sierra Nevada, was formed in this way, but the top was spared from basal sapping so that a nearly flat, tablelike summit remains of the original rolling, fluvial, pre-glacial topography [Guyton, 1998].
The preceding glacial forms all result more or less from gouging. One impressive form, however, is created instead by ice overriding and wearing down obstacles. This is the roche moutonnée, or “ fleeced rock, ” in French. When a sufficiently large, thick glacier reaches a hill, rather than dodging it, the glacier goes right over. What is left is an asymmetrical rock dome with a gentle, smoothed slope upstream and a steep slope downstream from where boulders have been plucked by the departing ice [Hill, 2006]. Yosemite is known for its roches moutonnées, such as Lembert Dome, whose present appearance was given it by the Tioga glacier.
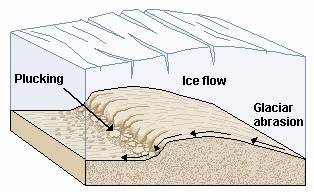
Diagram of glacial abrasion and plucking. Reproduced from http://fixedreference.org/2006-Wikipedia-CD-Selection/wp/g/Glacier.htm.
As glaciers move, they pick up a great deal of rock debris of all sizes known as till , either by freezing to it or by catching it when it falls from above. It is carried on, in, and under a glacier and can act as sandpaper on the bedrock beneath, making it smooth or even shiny. This is called glacial polishing [Hill, 2006]. Eventually, the till, having ridden the glacial “conveyor belt,” arrives at the melting tip of the glacier and is released from the ice. In this way, and also from a certain amount of plowing by the glacier, piles of unsorted rock debris, moraines , grow around its edges. Where they are preserved, these provide clues as to the paths of glaciers and the number of glacial events experienced by a particular spot. They also provide material that can be dated in attempt to determine when specific events took place. The most commonly seen types are probably end moraines , which form at the front when glaciers reach equilibrium and lateral moraines , which form at the sides.
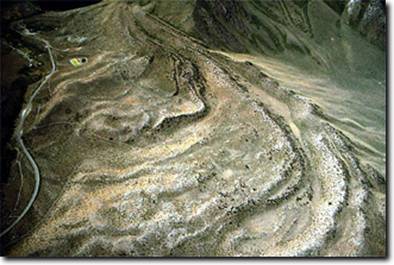
Lovely set of lateral moraines in the Sierra Nevada. Figure reproduced from http://vesr.ucnrs.org/pages/snarlgeol.html .
Medial moraines develop in the middle of ice streams from the merging of valley glaciers and their lateral moraines. Ground moraines form when glaciers melt and drop their loads of till in low, broad layers [Hill, 2006]. Lateral moraines are the easiest to spot in the Sierra Nevada and in many other places, as well. They are often quite large and are not as likely to be destroyed by subsequent advances as are other types which develop directly in the glacial path. These moraines often emerge from valleys at the bases of mountains. They have wedge-shaped cross sections and are typically found in curving pairs. Convict Creek, in the Eastern Sierra Nevada, has a nicely preserved set complete with sinuous depressions on at the outer edges where it looks as though liquid water ran down, presumably from melting in the zone of ablation. Convict Lake, set back toward the mountains perhaps a few hundred meters from the ends of the lateral moraines, is held in place by an end moraine. This type of set-up is found in many places in the Sierra Nevada and other mountain ranges which have experienced glaciation.
Pluvial lakes are lakes that grow due to increased precipitation and reduced evaporation. Since evaporation decreases along with decreasing temperature, these are typical conditions of an ice age. Indeed, “bathtub rings” far above the current levels of certain lakes in the Sierra Nevada suggest that they once held much more water. The Mono Basin, for example, held a succession of deep pluvial lakes during the late Pleistocene epoch known collectively as Lake Russell [Lajoie, 1968, as cited by Guyton, 1998]. Lajoie writes of “strand lines” at an altitude of 7,180 feet, the same altitude as the overflow channel into the Adobe Valley and subsequently the Owens River, indicating that the lake drained there. The present surface of the lake is at an altitude of less than 6,400 feet [Lajoie, 1968], and its water is extremely salty due to modern lack of drainage.
Constructing glacial history
For the most part, what we know about the history of glaciation in the Sierra Nevada and elsewhere has been pieced together by observation of physical features, like Mono Lake's bathtub rings, and making guesses about how they came to be there based on previous observations and interpretations. The accuracy of our interpretations is aided by a wide variety of dating procedures ranging from relative dating (e.g. using the fact that lava or ash is found above a layer of sediment to deduce that the glacial advance that deposited it took place before the eruption), to radiometric dating, which uses decay rates and ratios of certain naturally occurring radioactive isotopes to their decay products [http://en.wikipedia.org/wiki/Radiometric_dating]. In addition to its bathtub rings, the Mono Basin offers those studying glaciation particularly good moraines along some of its tributaries [Guyton, 1998], but also the sediment of its lakebed. Lakebeds, bogs, and ocean floors are valuable sources of glacial information because their sediments are less vulnerable to disruptions than those on dry land, and their records are therefore more complete and more detailed. Core samples from Mono Lake have suggested glacial advances from which no moraines are known, most likely having been destroyed by subsequent larger advances.
Francois Matthes was among the first to extensively study Sierra Nevada glaciation in the 1920s and 1930s. Much of his research was conducted in Yosemite, where he mapped moraines in detail, found “a four-fold record,” and concluded that there had probably been two earlier glaciations and one later one with a major fluctuation [Matthes, 1929]. He believed from its apparently fresh moraines and well-preserved glacial polish that the last glaciation there corresponded to the last glacial stage in the Rocky Mountains, and therefore the Wisconsin stage of the continental ice, so he applied to it the term Wisconsin [Matthes, 1929]. Matthes interpreted end moraines above Bridal Veil Meadow as an indication that during this period, the Yosemite glacier terminated within Yosemite Valley [1929]. The preceding stage had been more extensive, he thought, as lateral moraines from the Yosemite glacier of this period were to be found ten miles farther down the Merced Canyon around El Portal, the entrance to the park. Accordingly, he named this stage the El Portal . As evidence of a glaciation previous to this one, Matthes cited rows and groups of erratic boulders one or two hundred feet above the highest El Portal moraines. He concluded that these were the remnants of older moraines from which the finer material had been weathered away over time. From the facts that these boulders, although they were of durable rock, were greatly weathered, and that they had become quite rounded despite having only been moved about half a mile from where they originated, Matthes deduced that the advance that brought them there must have been very long ago. He named this stage Glacier Point after a typical grouping above that spot [1929].
Matthes' observations are fairly representative of findings throughout the Sierra Nevada, but research since has suggested more stages. His Wisconsin stage is thought to correspond to the Tioga and Tahoe and his El Portal probably to the Sherwin [Hayes]. The Glacier Point evidence is considered tenuous and is not widely accepted as corresponding to any one period [Hayes]. In 2004, Gillespie and Zehfuss wrote of eight named Pleistocene glaciations and stadials, which are, in decreasing order of age, McGee, Sherwin, Casa Diablo, Mono Basin, Tahoe, Tenaya, Tioga, and Recess Peak, as well as three or so Neoglacial advances ending with the Matthes (Little Ice Age), with additional advances evident in lake-sediment core samples. Interestingly, deposits from the first Pleistocene glaciation, the McGee, are found only atop divides—never in valleys. Glaciers cannot place till upon ridges, so what are now ridges must not have been at the time of the advance. Since this ground must have been at nearly its present elevation in order for glaciers to have formed, rather than the mountains having risen, the lowlands must have dropped [Guyton, 1998]. Such a scenario is consistent with the schema of north-south-oriented faulting and dropping of valleys throughout the Basin and Range Province, of which the Sierra Nevada are the westernmost range. There has been, and still is, much debate over the exact number of glaciations during the Pleistocene in the Sierra Nevada and when glacial events took place, but what glaciers have accomplished is certain. They, along with normal faulting, have made a sharp, rocky landscape out of one that was once gentle and rolling when governed by streams.
Conclusion
Over the last two centuries, we have learned a great deal about glaciers. In truth, it was not so very long ago that we thought of them as stationary, frozen masses. Now we are well aware of their mobility and their exceptional power to erode and alter their landscape, and we can even calculate the timing of the astronomical cycles which play such an important part in determining when glaciers form and when they melt. Nonetheless, questions remain. We still debate exact numbers of glaciations, their dates, and how they correlate between different parts of the world. Although cycles of Earth's orbit and rotation broadly explain the timing of glaciations in the Sierra Nevada and elsewhere, we have yet to design a model that works precisely. In fact, future climate change models will have a new factor to account for—a human factor. Gases and aerosols we release affect climate in complex ways, so far resulting in warming. Glaciers in the Sierra Nevada and much of the world are retreating, but increased snowfall in Antarctica is causing its central ice cap to grow [Botkin and Keller, 2007]. It is still unclear exactly what we should expect in coming years, but, by all indications, glaciers, the streams they feed, and therefore the surrounding regions, will be further affected by changes in temperature and precipitation. As for the Sierra Nevada Range, it is likely to become dryer, along with many other semi-arid regions [Botkin and Keller, 2007]. This is one compelling reason to study glaciers—by monitoring them, we can monitor the changes we are causing in Earth's climate, and this is something which needs very badly to be done.
References
Gillespie, Alan R., and Paul H. Zehfuss. Glaciations of the Sierra Nevada, California, USA . In: Ehlers, J., and P.L. Gibbard, ed. Quaternary Glaciations—Extent and Chronology: Part II: North America . Amsterdam: Elsevier; 2004: 51-62.
Guyton, Bill. Glaciers of California: Modern Glaciers, Ice Age Glaciers, Origin of Yosemite Valley, and a Glacier Tour in the Sierra Nevada: Vol. 59. California Natural History Guides. Berkeley: University of California Press; 1998.
Hayes, Garry. Glaciation of the Sierra Nevada. Available at: http://virtual.yosemite.cc.ca.us/ghayes/sierragla.htm . Accessed June 24, 2008.
Hill, Mary. Geology of the Sierra Nevada: Vol. 80. California Natural History Guides. Berkeley: University of California Press; 2006.
Matthes, F. E. Multiple Glaciation in the Sierra Nevada. Science [Serial Online]. 1929;70:75-76. Available at: http://www.jstor.org/stable/1652750 . Accessed: June 2, 2008.
Radiometric Dating. Wikipedia: The Free Encyclopedia. Available at: http://en.wikipedia.org/wiki/Radiometric_dating . Accessed June 24, 2008.
Tarbuck, E. J., and F. K. Lutgens. Earth Science . Columbus: Charles E. Merrill Publishing Co.; 1982.
Images
Arête. About.com: Geology. 2008. Photo by Bruce Molnia. Available at: http://geology.about.com/library/bl/images/blarete.htm courtesy of U.S. Geological Survey. Accessed June 25, 2008.
Cirque. About.com: Geology. 2008. Available at: geology.about.com/library/bl/images/blcirque.htm courtesy of Natural Resources Canada. Accessed June 12, 2008.
Glacier. 2006 Wikipedia CD Selection. Available at: http://fixedreference.org/2006-Wikipedia-CD-Selection/wp/g/Glacier.htm . Accessed June 25, 2008.
Metherel, Amanda. Secrets in the Ice: Glacial Movement Resulting in the Discovery of Ice Mummies. Available at: http://www.geocities.com/geoamanda/ . Accessed June 25, 2008.
Sierra Nevada Aquatic Research Lab. Copyright 2003-2008 University of California, Natural Reserve System. Available at: http://vesr.ucnrs.org/pages/snarlgeol.html . Accessed June 25, 2008.