Death Valley is on the edge of the Basin and Range Province , an area characterized by alternating north-south trending basins and ranges. These linear features are attributed to east-west crustal extension of the area, a process thought to be created by the interaction of plate tectonics. Extension stretches and breaks the crust apart, often along normal faults. The extended terrain produces uplifted horsts and down-dropped grabens. Though the processes creating the terrain are fairly recent and continue today, the rocks of Death Valley range from Precambrian to Recent and comprise all types of rock. Several geologic processes have occurred in this area, including deposition along both a passive and active margin; compression and extension of the crust; normal, reverse, strike-slip, and thrust faulting; volcanism and plutonism; and erosion of mountains and deposition of sediments in basins. During past glacial eras large lakes filled the basins, in addition to a smaller, more recent lake responsible for the development of the Death Valley salt pan.
Introduction
Death Valley is situated on the southwestern edge of the Basin and Range Province . This area is known for its alternating basins and ranges that run in a north-south trending direction and extends all the way from the Sierra Nevada's eastern flanks in eastern California through Nevada and into Utah [Hunt, 1975]. The development of these ranges
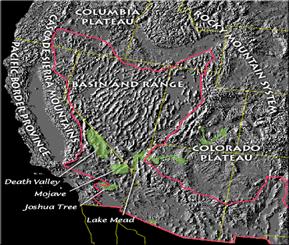
Figure 1. The Basin and Range Province , including Death Valley [http://geology.wr.usgs.gov/parks/deva/br.gif]
is attributed to east-west extension of the crust in the area, a process driven by plate tectonics that has been occurring for the past several million years and continues to be active today [Hunt, 1975]. Under the theory of plate tectonics, the earth's crust and uppermost mantle can be thought of as being broken up into a series of rigid plates that are slowly colliding with one another. As heat generated within the earth rises through the mantle, convection cells develop in which heated mantle material rises, cools, and then sinks. This cycle of rising and sinking causes a sort of conveyor belt upon which the plates move.
Crustal Extension
So what is causing extension of the crust in this area? One thought is that extension could be the result of the strike-slip movement of the Pacific plate against the western edge of the North American plate. The motion of the Pacific plate grinding past the North American plate could have possibly pulled on the North American plate, exerting tension and therefore stretching and pulling apart the area [Sharp and Glazner, 1997]. Another idea is that a smaller previously subducted plate has been subducted and consumed beneath the North American plate, causing disruption of the earth's crust [Sharp and Glazner, 1997]. It is possible that this scenario could have resulted from subduction of the Farallon plate, which had been involved with creating the Sierra Nevada to the west. There is no definitive answer either way, and it could be possible that both processes have played a role.
Subducting Slabs
Whether the crust has thinned and stretched because of heat related to underlying magma, or if extension of the crust has prompted magmatic activity by way of easy access to the surface continues to be debated [Sharp and Glazner, 1997]. Although this question has yet to be answered, some studies have made attempts to learn more about the processes involving plate tectonics and subduction. For instance, Severinghaus and Atwater [1990] put forth a hypothesis that the size and thermal state (hotness or coldness) of the slabs being subducted beneath the crust could perhaps be related to the extent of volcanism and tectonism that occurred during a geological timeframe, with warmer and smaller slabs having less impact on overlying processes than cooler or larger slabs.
Normal Faulting & Fault-Block Mountains
Rocks at or near the surface are relatively cool and therefore behave as a brittle solid, causing rock that is undergoing tension to fracture by way of normal faulting. This process causes the hanging wall to move downward relative to the footwall, forming structures known as horsts and grabens. It is the pulling apart of rock along these normal
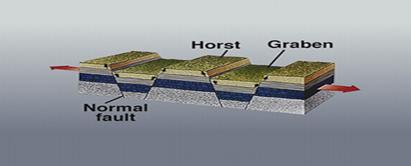
Figure 2. Normal faulting as a result of extension of the terrain [USGS].
faults that causes the alternating topography. The downward movement of the hanging wall creates a sinking basin, and the upward motion of the footwall pushes blocks of rock upward, creating uplifted blocks which are referred to as fault-block mountains. It is the development of these massive blocks of rock that have been uplifted and eroded to produce the mountains seen around Death Valley and the Basin and Range Province today. Snow and Lux [1999] analyzed Tertiary rocks in the Cottonwood Mountains, located on the northwestern edge of Death Valley, as well as in the Funeral Mountains on the northeastern edge, and concluded that the rocks from these mountain ranges were remarkably similar. Similar rocks located in separate mountain ranges indicated to them that the ranges had been located adjacent to one another prior to a period of extension that began occurring around 15 Ma, and had separated sometime between 10 and 6 Ma [Snow and Lux, 1999].
Death Valley Basin
As a result of crustal extension causing hanging walls to drop down and footwalls to move up, mountain-sized blocks of rock have been uplifted and tilted to the east, producing large, sediment-filled basins bordered by mountain ranges [Hunt, 1975]. McKenna and Hodges [1990] reconstructed a period of extension between the Panamint Range and the Black Mountains that occurred post-9 Ma, in which approximately 25 to 55 km of extension occurred over a period of 9 million years, producing an estimated rate of extension between 2.7-6.4 mm/yr. The movements that take place along these faults may be minute, yet displacement along the faults is great, since movement has been occurring for millions of years. As the rocks are pushed upward along the steep-angle faults, vertical and horizontal displacements along the faults can be significant. More technically, the downward motion of the Death Valley basin has actually produced what is known as the Death Valley half-graben, which, according to Knott [1999] was formed between two right-lateral-strike slip fault zones: the Furnace Creek and Death Valley fault zones.
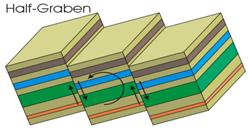
Figure 3. Uplift and Titling of Faulted Blocks. The Death Valley basin is a half- graben structure that has been filled with sediments transported from neighboring mountain ranges [http://facweb.bhc.edu/academics/science/harwoodr/GEOL101/Study/Images/HalfGraben.gif].
The down-dropping of the basins and uplift of fault-block mountain ranges has created an area with large variations in topographic relief. For instance, Telescope Peak in the Panamint Range rises nearly 3.5 km above the lowest point in Badwater Basin [McKenna and Hodges, 1990]. The geography of these tilted blocks situated along steep-angled normal faults results in steep, western-facing slopes coupled with gently sloping eastern faces [Hunt, 1975], such as is seen in Figure 4.
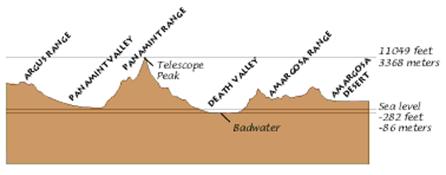
Figure 4. Cross section of Death Valley showing steep-sloped western mountain fronts along which steep-angled normal faults have uplifted the blocks; as well as gently-sloping eastern faces that have resulted from uplift and eastward tilting [http://b-thomas.com/dvnp/image2/ geology2.gif].
Geologic Record of Death Valley
Although many of the tectonic processes that have led to the formation of Death Valley are relatively young in age, some of the rocks in Death Valley are quite old. They span almost every geologic era and range in age from nearly two billion years to currently-forming salt deposits that could have formed only days or weeks ago. The ages and types of rocks along with their locations can been seen on the geologic map in Figure 5. Because of the largely intact geologic record available to geologists, a geologic history of the Death Valley can be deduced, including both tectonic and depositional environments throughout various points in time.
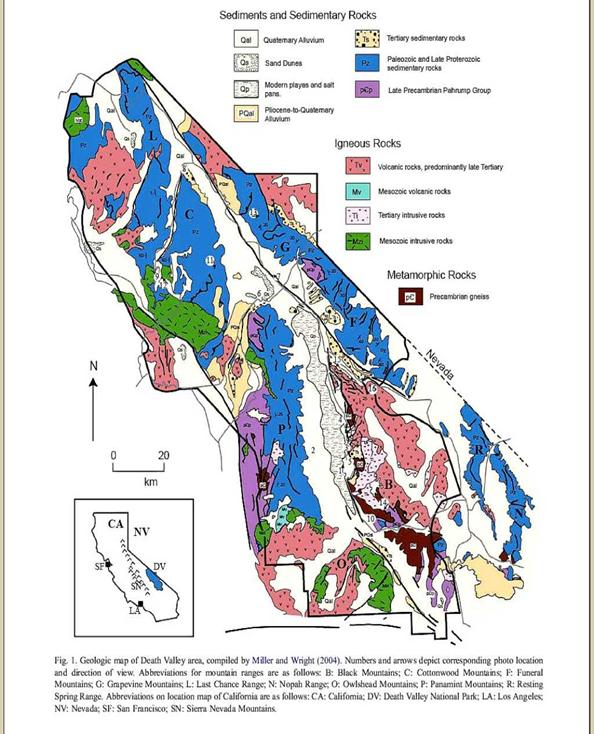
Figure 5. Geologic map of Death Valley showing bordering mountain ranges and locations of rock types. On the western side of Death Valley there are, from north to south, the Cottonwood, Panamint, and Owlshead Mountains. On the eastern side, from north to south, are the Grapevine, Funeral, and Black Mountains.[instruct.uwo.ca/.../cargo/indexcargo.htm].
Late-Precambrian to Early Cambrian
The oldest exposed rocks in Death Valley are from the middle Precambrian with an age of 1.8 billion years. They are severely metamorphosed volcanic and sedimentary rocks that have been altered into schists and gneisses. These rocks, once buried at great depth, have been uplifted and exposed in parts of the Black Mountains and Panamint Range and probably originated from an ancient volcanic mountain belt that had been covered with sand and mud deposits [USGS, Death Valley website]. Deeply buried and subjected to intense heat and pressure, these rocks have been severely altered from their parent rock. Overlying the crystalline basement are ancient sedimentary rocks consisting of conglomerates, sandstones, and mudstones. The rocks, known as the Pahrump Series, indicate that by near the end of the Precambrian about 1.3 billion years ago the metamorphic basement rocks had been uplifted and were shedding sediments into the shallow waters of the adjacent continental shelf.
Paleozoic Time
By about 550 million years ago the Death Valley area had switched depositional environments from sandy mudflats to a shallow sea of warm, clear water in which the main types of rock being deposited were carbonates, forming thick Paleozoic limestone and dolomite formations, which can be seen in the Grapevine and Cottonwood mountains to the northeast, as well as in Panamint Range in central Death Valley [USGS, Death Valley website]. Interrupting the periods of carbonate deposition were episodes of sand and mud deposition that are now evidenced by thick beds of quartzite that comprise the Stirling Quartzite, Wood Canyon Formation, and Zabriskie Quartzite Formations [USGS, Death Valley website]. These deposits were evidence of thrust-faulting and mountain-building processes that were happening north of the Death Valley area and would eventually be arriving in the area [USGS, Death Valley website].
Mesozoic Time
During late Paleozoic and Mesozoic time, about 225-65 million years ago, the Death Valley region switched from a passive to an active margin; a quiet continental shelf replaced by volcanism, mountain-building, and thrusting [USGS, Death Valley website]. At the time the continental North American plate was pushed up against the oceanic Pacific plate. Because the oceanic plate was denser and therefore less buoyant, it began subducting beneath the continental plate, forming a trench during the Triassic [USGS, Death Valley website]. As the plate was subducted some of it melted to produce magma and volcanism.
Compression
During the Jurassic and Cretaceous, magmatism related to the subduction was also producing chambers of magma beneath the volcanoes. The magma cooled at depth to crystallize into bodies of intrusive igneous rock called plutons. Most of the plutons formed what is now the Sierra Nevada, but a few intrusions of igneous rock reached Death Valley . These intrusions are younger than the Sierra Nevada but probably related to the same processes [Hunt, 1975]. They spread laterally into the Death Valley region along thrust faults, forming laccoliths. The thrusting developed as compressional forces created by the collision of the plates pushed blocks of rock eastward along low-angle reverse faults called thrust faults.
Extension
After 150 million years of tectonic activity the region experienced a period of relative quiescence during the Jurassic and Cretaceous, a time in which the rocks were elevated and exposed to erosion, converting high areas into lowlands while shedding and depositing sediments in low-lying areas [USGS, Death Valley website]. By the Miocene, volcanism and faulting had renewed, but this time as a result of extension rather than convergence and subduction [USGS, Death Valley website]. This renewed activity signaled the beginning of the tectonic processes that have shaped the Death Valley landscape seen today.
Cenozoic Time
During the Cenozoic there has been a well-recorded and complex history of magmatism and tectonism that has taken place to form the landscape as it is today. Cemen and Wright [1990] determined that Funeral Mountains , which contain both normal and thrust faults, have endured three separate extensional episodes at around 25 Ma, 20 Ma, and 14 Ma, with the 14 Ma extension producing the uplift of the fault-bounded blocks that form the current day mountain range. A separate study by Spencer [1990] allowed that in the late Cenozoic there were two separate and distinguishable tectonic settings. In the Mid-Miocene, from about 21-12 Ma, high-angle normal faulting was uplifting and eroding the Avawatz Mountains [Spencer, 1990]. From late Miocene to Pliocene north-south shortening was occurring, accompanied by folding and tilting of the Avawatz Mountains , eventually developing into a reverse-fault system [Spencer, 1990].
Central Death Valley
Death Valley is quite complex with regard to the development of its structure and associated faulting. Shear forces associated with the plate movement to the west caused strike-slip faults to develop on either side of Death Valley . The central part of Death Valley is a pull-apart basin between two right-lateral strike-slip faults, the Furnace Creek fault zone to the east and the Death Valley fault zone to the west. As both sides move sideways away from each other, the area in between is stretched and pulled apart, causing the valley to open up via a tensional gash in the basin [Sharp and Glazner, 1997]. At times faults have moved both laterally and vertically, creating a combination of strike-slip and normal faulting called oblique-slip faults [Geology of the Death Valley Area, Wikipedia].
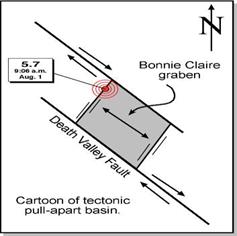
Figure 6. Illustration of a pull-apart basin. Pulling away on both sides produces a tensional gash. [www.seismo-watch.com/.../Graphics/Pull-apart.JPG].
Northern and Southern Death Valley
Northern and southern Death Valley are bounded on the east and west by normal fault troughs along which the valley dropped and mountains rose [Sharp and Glazner, 1997]. Additionally, localized extension is also occurring as a result of another fault, the Garlock Fault to the south, which is a left-lateral strike-slip fault. This fault is moving the Panamint Range westward, dropping the Death Valley graben to slip downward along the Furnace Creek fault system at the foot of the Black Mountains [Geology of the Death Valley Area, Wikipedia]. Death Valley 's remarkable terrain is thus the result of a series of faults that exhibit a rather complex behavior.
Sedimentary Fill
By Pleistocene time, about two million years ago, the current Death Valley topography had been developed [USGS, Death Valley website]. Because the basins in this region are formed from structural processes and are not the result of erosion, sediments that were shed into the valleys had nowhere else to go except to remain in the basin. The uplift of the mountain ranges provided a perfect mechanism for erosion of the rocky terrain and deposition of sediments in the basin, depositing as much as 2500 meters of gravel, sand, and mud in the Death Valley basin. However, Death Valley is 85 meters below sea level, and so for the basin to be able to remain below sea level and yet be filled with sediments it must be sinking at a rate faster than the rate of sediment deposition.
Pleistocene: Lake Manly
In the Pleistocene the climate was much cooler and wetter, and this produced glaciers in nearby mountain ranges and increased precipitation. Because the Death Valley basin is not the result of erosion and does not have an erosional outlet, incoming glacial meltwater that flowed into the basin had nowhere else to go, filling up the basin and creating large glacial-era lakes, collectively referred to as Lake Manly . These lakes were as deep as hundreds of feet [Hunt, 1975] and at its maximum level measured 600 feet deep and almost 100 miles long [Sharp and Glazner, 1997]. There is evidence of these lakes existing from between 240,000 years ago up until about 10,000 years ago [Sharp and Glazner, 1997]. They are evidenced by strandlines, which can consist of lake related features such as wave-cut cliffs, tufa deposits, or deposits of shoreline gravel [Sharp and Glazner, 1997]. However, in many places around Death Valley these features are not recorded or have been erased by weathering and erosion. Shoreline Butte and Mormon Pointe in the southern Black Mountains are good places to see strandlines [Sharp and Glazner, 1997].
Holocene: Recent Lake
During the Holocene, from about 5000 to 2000 years ago, there was another lake in Death Valley but much shallower, around 30 feet deep [Sharp and Glazner, 1997]. It is the evaporation of water from this lake that is responsible for the salt crust that covers the basin floor today. As this lake evaporated it left behind bands of salt of varying compositions [Sharp and Glazner, 1997]. Also, the floor of Death Valley tilted eastward, as shown by the eastern shoreline of this lake being some 20 feet lower than the western shoreline [Hunt, 1975].
Development of the Salt Pan
As the climate warmed, rainfall diminished and the lake began to evaporate. As water evaporated, minerals in the continually evaporating water became more and more concentrated until eventually the precipitated out of briny solution, covering nearly 200 square miles of lakebed [Hunt, 1975] with a salt crust ranging from about three to five feet thick [USGS, Death Valley website]. Sodium chloride is the most abundant salt in Death Valley , but there are other varieties that precipitate here as well, with carbonates having the lowest solubility and thus precipitating first, followed by sulphates and lastly chlorides [Hunt, 1975].
Chemical Zoning
These different types of salt are layered throughout the basin in concentric zones reflecting the solubility of the water at the time of deposition [Hunt, 1975]. Sodium chloride is the most soluble, and therefore is precipitated last and occupies the vast majority of the basin from the center on out. Sulphates are deposited in a discontinuous ring around the chloride zone, followed by carbonates, which are deposited in places around the edge of the basin.
Polygonal Structures
Contributing to the mysterious nature of Death Valley is a rather curious structural feature of the salt crust. Contraction of the salt pan during dry periods forms polygonal structures that form by outward growth around the edges of slabs of salt comprising the Death Valley floor [Sharp and Glazner, 1997]. Beneath the salt lies a thick layer of moist silt and mud. It is believed that drying and contraction of the mud produces mud cracks around which salt water seeps up through the soil [Hunt, 1975]. As it does so, the water evaporates leaving behind salt crystals that grow in and around the cracks. The process repeats itself until eventually raised polygonal features have formed. As long as no sporadic floods reach these features, the salt comprising these features is not in peril of being dissolved and re-precipitated somewhere else. Crystallization at the edges of the polygons causes the slabs to grow outward, often pushing up against and thrusted over one another [Sharp and Glazner, 1997].
Conclusion
The Death Valley area is an area of extremes. It is one of the hottest and driest regions on the North American continent, yet as little as 2000 years ago stood a lake 30 feet deep. The geologic record ranges between ancient metamorphic rocks that are almost two billion years old to evaporites that are still currently forming today. High, rocky, mountain ranges that are being uplifted are interrupted by sinking, sediment-filled basins. The area is currently undergoing extension, yet in the past it lay witness to scenes of subduction and compression, volcanism and plutonism. Strike-slip, normal, and oblique-slip faults all work together to tear the terrain apart. And the story remains incomplete, as geologists continue to work today to decipher this region's cryptic past. For anyone interested in earth processes and how the earth has come to arrive at such a unique existence, Death Valley offers the perfect classroom to study and learn about past and present earth processes which at times can be quite complex and dynamic, and will continue to operate for many millions of year to come.
References Cited
Cemen, I. , and Wright, L. A., 1990, Effect of Cenozoic extension on Mesozoic thrust surfaces in the central and southern Funeral Mountains , Death Valley , California , in Wernicke, B. P., ed., Basin and Range extensional tectonics near the latitude of Las Vegas , Nevada : Boulder , Colorado , Geological Society of America Memoir 176.
Geology of the Death Valley area. (2008, May 18). In Wikipedia, The Free Encyclopedia. Retrieved May 30, 2008, from http://en.wikipedia.org/w/index.php?title=Geology_of_the_Death_Valley_area&oldid=213168328
Hunt, C. B., 1975, Death Valley : Geology, ecology, archaeology: Berkeley, University of California Press, 234 p.
Knott, J. R., Sarna-Wojcicki, A. M., Meyer, C. E., Tinsley, J. C., III, Wells, S. G., and Wan, E., 1999, Late Cenozoic stratigraphy and tephrochronology of the western Black Mountains piedmont, Death Valley, California: Implications for the tectonic development of Death Valley, in Wright, L. A., and Troxel, B. W., eds., Cenozoic Basins of the Death Valley Region: Boulder, Colorado, Geological Society of America Special Paper 333.
McKenna, L. W., and Hodges, K. V., 1990, Constraints on the kinematics and timing of late Miocene-Recent extension between the Panamint and Black Mountains, southeastern California , in Wernicke, B. P., ed., Basin and Range extensional tectonics near the latitude of Las Vegas , Nevada : Boulder , Colorado , Geological Society of America Memoir 176.
Severinghaus, J., and Atwater , T., 1990, Cenozoic geometry and thermal state of the subducting slabs beneath western North America , in Wernicke, B. P., ed., Basin and Range extensional tectonics near the latitude of Las Vegas , Nevada : Boulder , Colorado , Geological Society of America Memoir 176.
Sharp, R. P., and Glazner, A. F., 1997, Geology underfoot in Death Valley and Owens Valley: Missoula , Montana , Mountain Press, 321 p.
Snow, J. K., and Lux, D. R., 1999, Tectono-sequence stratigraphy of Tertiary rocks in the Cottonwood Mountains and northern Death Valley area, California and Nevada, in Wright, L. A., and Troxel, B. W., eds., Cenozoic Basins of the Death Valley Region: Boulder, Colorado, Geological Society of America Special Paper 333.
Spencer, J. E., 1990, Late Cenozoic extensional and compressional tectonism in the southern and western Avawatz Mountains , southeastern California , in Wernicke, B. P., ed., Basin and Range extensional tectonics near the latitude of Las Vegas , Nevada : Boulder, Colorado, Geological Society of America Memoir 176.
USGS. Death Valley National Park Virtual Field Trip. (2004, January 13). Retrieved May 30, 2008 from http://geomaps.wr.usgs.gov/parks/deva/devaft.html