ABSTRACT
Glaciers are unlike anything else in this universe. They have the ability to transform the Earth's surface in ways unimaginable—literally tearing up the land from where it once resided and carrying it to its final resting place possibly miles away…or acting as Mother Nature's sand paper leaving behind a more suitable polished surface. Yosemite National Park 's magnificent landscape is the result of a series of glaciations beginning millions of years ago: the Tioga, Tahoe, and Pre-Tahoe. Each glaciation scoured the land and exerted upon it a variety of glacial forces that created Yosemite as we know it today. The Tioga glaciation began roughly 60,000 years before present and peaked around 20,000—reaching as far into the park as Bridalveil Meadow. The Tahoe glaciation was considerably larger then the Tioga and covered a total of 33 miles, peaking roughly 130,000 years before present. The Pre-Tahoe serves as the oldest glaciation and encompasses glaciations that are older than 200,000 years before present. The Sherwin glacier, which is considered to be the most extensive and influential glaciation in Yosemite 's history, occurred roughly 800,000 years before present and within the Pre-Tahoe glaciation. Evidence of glaciation can be found throughout Yosemite National Park in the form of potholes, moraines, glacial polish, and erratics. Furthermore, the evolution of landforms such as Yosemite Valley, Tuolumne Meadows, Bridalveil Fall, and Yosemite Falls can only be attributed to glacial forces.
Overview of Yosemite 's History
Yosemite Discovered
Yosemite National Park has long since been known for its beautiful sights, cascading waterfalls, and sheer rock faces to people around the world—including tourists, avid rock climbers, and scientists. Yosemite National Park , located within the greater Sierra Nevada mountain range, was officially discovered when the American Indians embraced the valley's territory to evade the United States Mariposa Battalion in 1851, according to Earth Science Professor James S. Aber [2004]. Major James Savage, who led the Mariposa Battalion in this endeavor, is credited to have discovered the valley [Aber, 2004]. However, it is likely the valley was found much earlier by American Indians or even explorers such as those led by Joseph “Snowshoe” Walker in 1833 [Aber, 2004]. Thanks to influential people and organizations, such as United States' President Abraham Lincoln and the United States Geological Survey, Yosemite Valley has been magnificently preserved and remains relatively unharmed by the human hand; thus, allowing scientists to study its various naturally occuring intricacies. One intricacy hotly debated around the turn of the 20 th century was Yosemite 's geological history and how it came to be.
Battle of the Scientists
Josiah Whitney was the head of the Geological Survey of California and first visited Yosemite in 1863, where he was convinced Yosemite was the result of major faulting processes [Aber, 2004]. According to Whitney, there were two major faults running around the valley that eventually gave way and dropped the valley down to where it is today [Aber, 2004]. Whitney believed that glaciers had little to no impact in shaping Yosemite 's landscape [Aber, 2004]. On the other hand, John Muir, a now well-known, early environmentalist, came to a different conclusion after years exploring, studying, and living in Yosemite Valley [Aber, 2004]. Muir believed that glaciers played a monumental role in forming Yosemite 's landscape [Aber, 2004]. John Muir had not been formally educated in geology but had spent time in Alaska learning the effects glaciers can have on the Earth's surface; using this information, he was able to go into extensive detail about the formation of Yosemite National Park [Aber, 2004].
By 1930, the results of Yosemite's geological history were formally published, after an eighteen-year study of Yosemite by the United States Geological Survey [Aber, 2004]. The two leading geologists on the study, Fracois Matthes and Frank Calkins, came to the conclusion the valley had been formed by a series of glaciers [Matthes, 1930]. While glaciers have taken a major role in the evolution of Yosemite National Park , other geological forces have taken their toll on the landscape including: plate tectonics, major faulting, joints, and other forms of erosion such as water. Although not covered in this paper, these forces should be given due credit for their various roles in the formation of one of our nation's most beautiful national parks.
A Brief Overview of Geological Terms
General Glacial Terms
Before jumping more deeply into the geological past of Yosemite Valley , I'd like to go over a few key concepts regarding geological terms that will be used in this paper. According to Lutgens and Tarbuck [2000], co-authors of the market's best selling educational earth science book, a glacier is a thick mass of ice that generally forms in areas previously inhabited by streams. Glaciers continue to grow as snow accumulates at the top of the glacier and ice melt recrystallizes [Lutgens and Tarbuck, 2000]. Glaciers are different than ice sheets , which are much larger and are sometimes referred to as continental ice sheets since they cover areas such as Antarctica and Greenland [Lutgens and Tarbuck, 2000]. Ice sheets have a different effect upon the land than glaciers, making it important to distinguish between the two terms.
As temperatures, precipitation, and other weather variables vary from day to day and climates continue to change, glaciers are continually undergoing change and each has a budget on which to work. According to N. King Huber, a well-known expert in Yosemite geology, the glacial budget refers to the balance between the accumulation at the top of the glacier from snowfall and the loss at the bottom of the glacier from melting and evaporation, also known as ablation [1987]. If there is more accumulation than loss, the glacier advances, growing and extending down-valley, until it reaches equilibrium [Lutgens and Tarbuck, 2000]. On the other hand, if the loss is greater than the accumulation, the glacier will retreat, essentially melting away at the bottom and “retreating” up-valley [Lutgens and Tarbuck, 2000].
As a glacier reaches a thickness of roughly 100 feet, the weight takes its toll and glaciers begin to move, sometimes moving even before reaching this critical mass on a steeper slope [Huber, 1987]. According to Huber, glaciers generally begin to melt at a faster rate as they descend the slope, since temperature increases as elevation decreases. The point at which a glacier begins to melt at a faster rate is called the glacier's firn limit , or equilibrium-line altitude (ELA), where annual loss exceeds annual accumulation [Huber, 1987]. According to Huber, the ELA can be over 1000 feet lower in elevation on the shady side of the mountain than the ELA on the sunny side. This explains why the “north face” of the mountain produces much harsher conditions for animals, climbers, and skiers [Huber, 1987].
Glacial Erosion
As one would assume, when such a large mass of ice moves across the surface of the earth it generally causes damage along the way by eroding the land on which it “lives.” According to MacGregor et al. at the University of California-Santa Cruz's Department of Earth Sciences, erosion is expected to increase heavily upon the glacier passing the ELA. At the ELA, both sliding speed and water-pressure fluctuations generally reach their maximum and have the greatest impact on the rate of erosion and quarrying rate (respectively) [MacGregor et al., 2000]. According to MacGregor et al., the average rate of erosion for a “typical” glacier exposed to variety of climates is 1 mm per year [2000]. While this may seem insignificant, glacial erosion over a period of time can invoke an enormous amount of change on the earth's surface. Two common sources of glacial erosion are plucking and abrasion . Ice melt from the glacier seeps directly into the rock on which it sits and then freezes, expands, and causes the bedrock to break apart allowing the glacier to “pluck” the rock up and carry it along with other glacial debris [Lutgens and Tarbuck, 2000]. Abrasion occurs as the glacier moves along the rock's surface, scraping, and grinding—possibly leaving behind a polished surface or maybe striations. Glacial striations provide evidence of the direction of flow and help geologists reconstruct the glacier's previous path [Lutgens and Tarbuck, 2000]. This allows geologists to understand the extent and expanse of glaciation in the area. Abrasion is usually seen on a smaller scale, while plucking has been known to remove large portions of rock known as erratics , which are left behind as the glacier begins to melt [Huber, 1987].
Glacial Landforms
Glacial erosion is also known to create a number of distinct landforms such as glacial troughs, hanging valleys, cirques, arêtes, and horns. Glacial troughs are U-shaped valleys formed by glaciers as they widen, deepen, and straighten previously V-shaped valleys as they move [Lutgens and Tarbuck, 2000]. Glaciers typically have a main glacier, trunk glacier , and several tributary glaciers that are thinner and less imposing on the earth's surface [Lutgens and Tarbuck, 2000]. Therefore, hanging valleys result when tributary glaciers erode the land less than trunk glaciers leaving tributary paths to “hang” above the trunk valley [Lutgens and Tarbuck, 2000]. According to Lutgens and Tarbuck, cirques are “bowl-shaped depressions that have precipitous walls on three sides but are open on the down valley side.” Cirques are very prominent because this is where the glacier grows, accumulating snow and forming ice in the same spot for a long period of time [Lutgens and Tarbuck, 2000]. Horns (sharp peaks) and arêtes (sharp-edged ridges) are created when cirques are exposed to a great amount of erosion over a period of time. When a single peak is surrounded by cirques on all or many of its sides, it continues to erode until there is a large, single peak that looks much like a “horn” of an animal [Lutgens and Tarbuck, 2000]. Arêtes are formed in a similar manner, but the cirques are only located on both sides of a mountain edge and they erode until there is a very sharp ridgeline [Lutgens and Tarbuck, 2000].
Glaciers are also known to leave behind less commonly known features such as potholes and glacial polish . As glaciers begin to melt, streams of ice-melt flow beneath the glacier carrying debris [Huber, 1987]. Potholes , shown in Figure 1, are formed when rock sediments in the sub-glacial stream are swirled around in the same spot for a period of time by the force of the stream [Huber, 1987]. This exposes the surface to continual erosion and eventually erodes away a portion of the rock surface that looks much like a bowl [Huber, 1987]. Glacial polish is a clear indication of glaciation and is the result of glacial debris, such as sand and pebbles, grinding the surface of which it flows over acting as sand paper and leaving behind a polished surface [Huber, 1987]. All of these landforms and features are characteristic of glacial erosion and can be seen in areas that are currently glaciated as well as areas that were once glaciated—such as Yosemite .
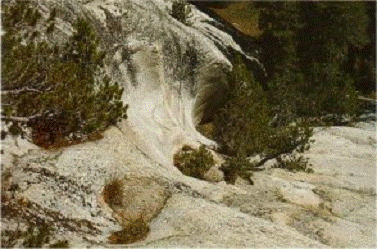
Figure 1: Pothole on Pothole Dome in Yosemite National Park [Huber, 1987] (Exact Reproduction)
Glacial Deposition
While glaciers are responsible for a considerable amount of erosion, they are just as well known for their deposition. As glaciers move along, they accumulate large amounts of debris such as: sand, rocks, clay, and boulders. This unsorted mixture of debris is commonly called glacial till and is responsible for creating glacial landforms called moraines [Huber, 1987]. Moraines are one of the most common glacial landforms and can be divided into lateral, medial, end, and ground moraines. According to Lutgens and Tarbuck, lateral moraines are ridges of till formed on parallel sides of the glacier [Figure 2], whereas medial moraines are formed when lateral moraines merge together [Figure 3]. According to Huber, medial moraines are evidence that glaciers really do move, as there would be no way to produce these stripes of debris unless the glacier moved down valley.
End moraines are formed as glaciers reach a state of equilibrium, neither growing nor receding. In this state of equilibrium, the glacier remains stationary while the glacier transports various amounts of debris down valley [Lutgens and Tarbuck, 2000]. As the debris flows down valley, it winds up at the end of the glacier and forms the end moraine, growing larger as the glacier remains in equilibrium [Lutgens and Tarbuck, 2000]. When the glacier begins to recede, the sediment is deposited at various places along the way filling in depressed areas and covering up existing streambeds. This is known as a ground moraine . According to Lutgens and Tarbuck, glaciers can produce a series of end and ground moraines before the glacier has completely vanished. Geologists must be able to determine the difference between terminal moraines , those formed as the glacier terminates, and recessional remains , those formed before the termination of the glacier [Lutgens and Tarbuck, 2000].
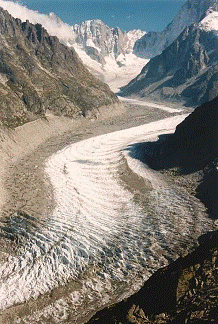
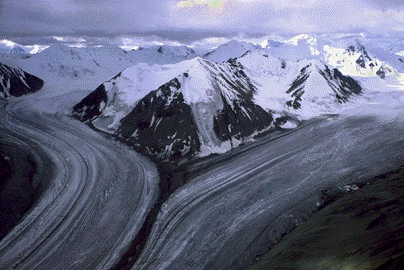
Figure 2 (left): Lateral moraine in Mer de Glace, France caused by an alpine glacier that has been deteriorating throughout the 20 th century [ Hubbard et al., 2004] (Exact Reproduction)
Figure 3 (right): Medial moraine formed when two glaciers merged together [“Rivers of Ice”, Kluane National Park ] (Exact Reproduction)
Glacial deposition also comes in the form of glacial erratics [Figure 4]— rock fragments ranging in size from small to extremely large that have been “plucked” from the bedrock and moved by glacial forces to other areas [“Glacial Erratic”]. On a broader scale of glacial deposition, glaciers are known to leave behind kettles— small lakes located in depressions of land which were filled in with ice-melt as the glacier finally melted [Huber, 1987]. Overall, these forms of deposition are typical of glaciated regions and can be seen in glaciated areas such as Yosemite National Park .
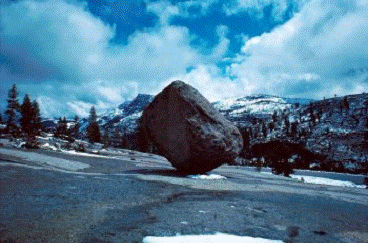
Figure 4: Glacial erratic in Yosemite National Park located near Olmstead Point [Huber, 1987] (Exact Reproduction)
Yosemite's Glacial Past
Glacial Episodes
Ever since John Muir's initial exploration and study of Yosemite National Park , geologists have been interested in the history of the valley, especially its geological, glacial past. Many geologists have studied this matter and all would agree Yosemite has been glaciated on at least three separate occasions starting roughly three million years before present [Aber, 2004]. Francois Matthes, a prominent geologist who participated in the eighteen-year study of Yosemite with the United States Geological Survey, coined the glaciers Wisconsin, El Portal, and Glacier Point, from youngest to oldest respectively [Matthes, 1930]. However, another geologist studying the eastern Sierras in roughly the same time period, Eliot Blackwelder, noted four glaciers that he named Tioga, Tahoe, Sherwin, and McGee [Huber, 1987]. While there have been numerous terms for the glaciers in the Yosemite Valley, the Pre-Tahoe , Tahoe , and Tioga , from oldest to youngest respectively, are now considered by geologists to be the correct glacial episodes in Yosemite National Park [Huber, 1987]. We will use these terms hence forth to describe Yosemite 's geological, glacial past.
While all three of these glaciers encompassed areas outside of Yosemite, we will use specific definitions to describe how geologists distinguish the glaciations inside Yosemite National Park —since definitions of the greater glaciations are not sufficient. According to Huber, the youngest glaciation, Tioga, is distinguished by geologists as “unweathered till consisting of fresh granitic boulders and loose, porous gravel and sand, making sharp-crested moraines that have closely spaced boulders on their upper surfaces [1987].” The most important part of the definition is the use of the word “unweathered.” Since the Tioga is the youngest, it has not been exposed to as much erosion as previous glaciers. The rest of the definition is useful when looking into more specific details of glaciation.
Furthermore, Huber states the intermediate glaciation, Tahoe, is distinguished as “subdued moraines whose buried grandiorite boulders have commonly disintegrated at least partly to gnus. The summits of these moraines tend to be rounded, and exposed boulder along their crests are generally sparse [1987].” Again, the key words in this definition relate to the amount of erosion the glacial evidence has been exposed to causing the landforms to be subdued and rounded. Since the Tahoe glaciation happened before the Tioga, it has been exposed to a higher degree of weathering causing its moraines to be less extreme. Finally, the oldest glaciation, Pre-Tahoe, is described by Huber as “outside the ice limits defined by the intermediate moraines, a few glacial erratics, commonly perched, and patches of formless glacial till give evidence of at least one earlier ice advance more extensive than the glaciers that deposited the intermediate moraines [1987].” The Pre-Tahoe glaciation has been exposed to the elements for hundreds of thousands of years, making it very difficult to find evidence of its existence in the park.
Evidence for all three glaciations can be seen at Harden Lake , near the upper valley and Tuolumne River , where all three glaciations are known to have reached [Huber, 1987]. At Harden Lake , terminal moraines from Tioga lie within less prominent terminal moraines from the Tahoe, which are surrounded by large erratics typical of the Pre-Tahoe glaciation [Huber, 1987]. Each of the Yosemite glaciations will be described in detail in the following portions.
Yosemite's Glaciers Defined
Tioga Glaciation
According to Huber, roughly 30,000 to 60,000 years before present the climate began to cool (not unusual as climate fluctuations across the globe are quite common and follow a pattern of warming and cooling over millions of years), and this is when the Tioga glaciation began to form [1987]. The glacier grew over thousands of years and reached its maximum around 15,000 to 20,000 years before present—covering much of the Sierras and reaching areas such as Yosemite, the Mono lowlands, and San Joaquin River [Huber, 2004]. Tioga's terminal moraine in Yosemite lies within the Bridalveil Meadow—evidence that, at its peak, it only reached this far into the park [Huber, 2004]. However, at its peak the Tioga glaciation reached a maximum thickness of 2000 feet in Little Yosemite Valley [Schaffer, 2004]. Furthermore, there were a number of smaller glaciers that were not part of this larger system during Tioga's time period, which grew in cirques left behind by older glaciers, and played various roles in shaping Yosemite 's beautiful landscape [Huber, 1987].
Tahoe Glaciation
The Tahoe glaciation is thought to have peaked roughly 130,000 years before present but may have begun as early as 200,000 years before present [Huber, 2004]. While the Tahoe glaciation in Yosemite was slightly bigger than that of the Tioga glaciation, the Tahoe glaciation, which covered the greater Sierra landscape, was much larger than that of the Tioga [Schaffer, 2004]. Evidence of the size of the Tahoe glaciation can be seen in outlying areas where Tahoe moraines lie beyond the Tioga moraines—proving it was in fact larger [Huber, 1987]. Due to this evidence in the greater Sierra area and numerous studies, geologists believe the Tahoe glaciation reached further than the Tioga in Yosemite ; however, there is no clear evidence of this due to the lack of terminal Tahoe remains [Huber, 2004]. According to Schaffer, the Tahoe glaciation was roughly 33 miles long and up to 1600 feet thick at Glacier Point, whereas the portion reaching the Grand Canyon of Toulumne River outside of the park was approximately 58 miles long and up to 3500 feet thick near Hetch Hetchy's Dam [Schaffer, 2004].
Pre-Tahoe Glaciation
Glaciations occurring before 200,000 years ago and, subsequently, before the Tahoe glaciation, are known as the Pre-Tahoe glaciations [Schaffer, 2004]. These glaciations may have begun as early as 2.5 million years before present and most likely include more than 36 glaciations, according to Schaffer. The Sherwin glaciation is one of the most commonly known glaciations in Yosemite history and can be dated to roughly 900,000 to 800,000 years before present—thus making it part of the Pre-Tahoe glaciations [Schaffer, 2004]. The Sherwin glaciation is considered to have lasted over 300,000 years—lasting so long it is now widely considered to be the most extensive glaciation in Yosemite and most likely responsible for shaping and creating the valley in the Merced River Canyon [Huber, 2004]. While Pre-Tahoe glaciations are attributed to shaping much of Yosemite, evidence of Pre-Tahoe glaciation in Yosemite National Park is sparse [Schaffer, 2004]. According to Huber, the main evidence for Pre-Tahoe glaciation lies among the various erratics located near the park in the eastern upland valley as there is no evidence of any terminal or recessional moraines [1987]. While there is no terminal moraine for this glaciaton, Huber believes it may have reached to the town of El Portal but no further—as the canyon begins to take the form of a V-shape and meander, not common glacial landscape characteristics [2004]. Despite the lack of clear evidence for Pre-Tahoe glaciation, geologists still believe it was the largest of all Yosemite glacations and is illustrated in Figure 5. At the height of the Sherwin glaciation, the highest peaks such as Mount Dana and Mount Conness were the only ones to remain above ice level [Huber, 2004].
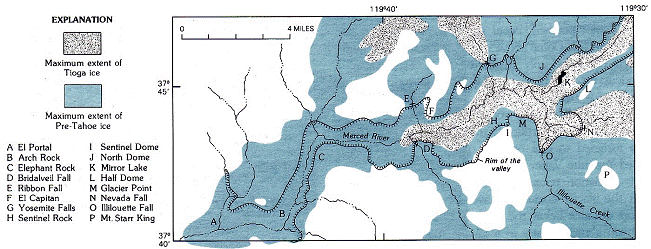
Figure 5: Pre-Tahoe glaciation (blue) reaches much more of Yosemite than that of the Tioga glaciation (grey) [Huber, 1987] (Exact Reproduction)
Neoglaciation
While the Tioga glaciation was the last to cover a great amount of the Sierra landscape, there have been a number of glaciers throughout the Sierras beginning around 2,500 years before present—representing Neoglaciation [Huber, 2004]. Roughly 10,000 years before present, as the Pleistocene came to an end, whatever was left of the Tioga glaciation would have began to deteriorate and disappear by 5,000 years before present [1987]. Global temperatures continued to rise and fall throughout the next 1000 years with the last major downfall in temperatures occurring in 1850—the same time period glaciers in the Alps began to advance [Huber, 1987]. As previously mentioned, John Muir was conducting his research in the Sierras shortly after that time period and, according to Huber, geologists believe that the glaciers would have been at their height while he was there. Glaciers seen today in the Sierras are much too small to produce the sort of landforms created by the Tioga, Tahoe, and Pre-Tahoe glaciations. Much of the erosion taking place in the valley today is the result gravity in the form of avalanches, rock falls, and flowing water, which will not be discussed in detail.
Yosemite's Glacial Wonders
Glacial Valleys—Yosemite Valley, Tuolumne Meadow
As seen in Figure 6, Yosemite Valley portrays the U-shaped valley typical of glaciated regions. The Sherwin glaciation is typically held responsible for creating this shape, as it was the most extensive and longest lasting glaciation in the valley [Huber, 2004]. During the Sherwin glaciation, only the very top portion of El Capitan would have remained above the ice level and would be able to withstand the forces of glaciation, because it lacked any major zones of weakness [Aber, 2004]. According to MacGregor et al., multiple glaciations in the same area does not equate to a deeper valley. Once a glaciation has taken place on the landscape, the next glaciation to take place there will be under a completely different set of circumstances—essentially changing the erosion rate and applying different forces to the land [MacGregor et al., 2000]. Therefore, it is reasonable to assume that the Sherwin glaciation is responsible for this U-shaped valley—later glaciations most likely only refining the landscape [MacGregor et al., 2000]. In areas that were not glaciated, such as the Merced River Canyon east of El Portal, valleys retain the V-shape form typical of non-glaciated areas—proving the U-shape is not common throughout the region [Aber, 2004].
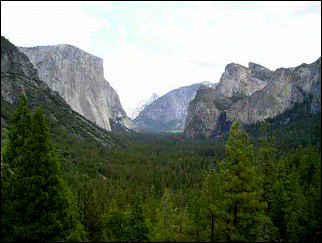
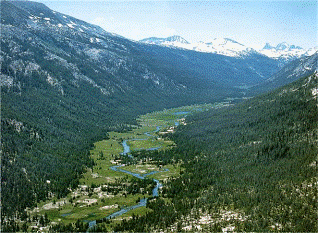
Figure 6 (left): Yosemite Valley with El Capitan to the left and Bridalveil Falls to the right—showing typical U-shape of glaciated valleys [Aber, 2004] (Exact Reproduction)
Figure 7 (right): Lyell Fork of the Tuolumne River in the Tuolumne Meadows [Huber, 1987] (Exact Reproduction)
Tuolumne Meadows, as seen in Figure 7, takes form as the quintessential U-shaped valley and provides us with further compelling evidence of glaciation in the Yosemite. While the bottom of the valley has taken a flatter appearance over the years, it should be remembered that it was created by glacial forces. Much of the sedimentation taking place and filling in the once U-shaped valley took place thousands of years ago—literally laying the ground work for today's meadow to flourish [Huber, 1987].
Hanging Valleys—Bridalveil Fall
As mentioned earlier, hanging valleys are typical representations of glacial landforms as they are formed when tributary glaciers erode the land far less than that of the trunk glacier. Trunk glaciers are much more dense than those of their tributary glaciers and, therefore, are able to erode the landscape to a greater extent [Aber, 2004]. According to MacGregor et al., hanging valleys are created more rapidly as the ratio between tributary and trunk discharge increases [2000]. Tributary paths are then left hanging over the main trunk valley, creating landforms such as Bridalveil Fall. As you can see in Figure 8, Bridalveil Fall flows directly out of the mouth of a tributary glacial valley down to the much deeper trunk valley. Bridalveil Fall was formed during the Sherwin glaciation and provides sightseers today with a spectacular waterfall cascading down a total of 620 feet.
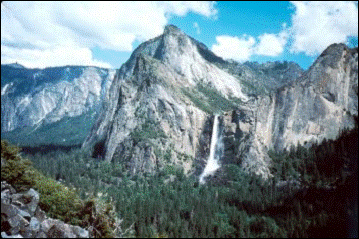
Figure 8: Bridalveil Fall is the result of a hanging valley—formed as tributary glaciers eroded the land less than that of the trunk glacier during the Sherwin glaciation [Huber, 2004] (Exact Reproduction)
Yosemite Falls
Yosemite Falls most likely came to be roughly 130,000 years before present at the height of the Tahoe glaciation [Aber, 2004]. While Yosemite Falls [Figure 9] is the result of a hanging valley, it did not produce such magnificent sights seen today before this time period.
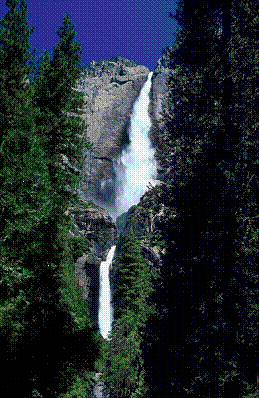
Figure 9: Yosemite Falls showing the Upper Fall, Middle Cascades, and Lower Fall [Pinkers] (Exact Reproduction)
Prior to 130,000 years before present, Yosemite Creek flowed in a different direction and Yosemite Falls was only active during the spring run-off season at a far lesser extent [Huber, 2004]. According to Huber, during the Tahoe glaciation, Yosemite Creek was diverted from its original path by a portion of glacier to the path seen today—creating the Yosemite Falls we have come to love and admire. During the Tahoe glaciation, Yosemite Creek's original path was obstructed by glacial debris, and the ice melt found an alternative path of least resistance—continuing to flow through the new channel for thousands of years and carving the path seen today [Huber, 2004]. Thanks to the Tahoe glaciation, Yosemite Falls is the world's fifth tallest waterfall and is made up of three falls (Upper Fall, Middle Cascades, and Lower Fall)—dropping a total of 2425 feet and flowing at a measurement of roughly 300 cubic feet per second [“Yosemite Falls…”].
Conclusion
The awe-inspiring and magnificent Yosemite National Park is the result of millions of years of Mother Nature at work—slowly creating the impressive landscape we know and enjoy today. Thanks to numerous geological studies of the area, it is now widely accepted three major glaciations took place in Yosemite —Tioga, Tahoe, and Pre-Tahoe—each taking their own toll on the land. Wonders such as Yosemite Valley, Tuolumne Meadows, Bridalveil Fall, and Yosemite Falls are the result of these glaciers and can only be attributed to these powerful forces—as nothing else could create such beautiful and dramatic landforms.
Works Cited
Aber, James S. " Yosemite Valley : a Glacier Carved Jewel." 25 Nov. 2004. Earth Science Department, Emporia State University . 22 Dec. 2007 <http://www.emporia.edu/earthsci/student/gunther2/yosemite.html>.
"Glacial Erratic." Geology Dictionary. 24 Dec. 2007 <http://www.alcwin.org/Dictionary_Of_Geology_Description-75-G.htm>.
Hubbard, Bryn, Owain Bayley, David Chandler, and Michael Hambrey. "Valley Glacier Dynamics and Structure." 2004. Centre for Glaciology, the University of Whales. 21 Dec. 2007 <www.aber.ac.uk/~glawww/alps.htm>.
Huber, N. King. "Geologic Story of Yosemite Valley : In the Footsteps of Francois E. Matthes." USGS: Science For A Changing World. 13 Jan. 2004. United States Geological Survey. 22 Dec. 2007 <http://wrgis.wr.usgs.gov/parks/yos/topobk.html>.
Huber, N. King. The Geologic Story of Yosemite National Park . 1987. Yosemite National Park . 21 Dec. 2007 <http://www.yosemite.ca.us/library/geologic_story_of_yosemite/final_evolution.html>.
Lutgens, Frederick K., and Edward J. Tarbuck. Earth: An Introduction to Physical Geology . 9th ed. Upper Saddle River : Prentice Hall, 2000. Glaciers, Deserts, and Wind. 21 Dec. 2007 <http://wps.prenhall.com/esm_tarbuck_escience_9/3/1011/258966.cw/index.html>.
MacGregor, K.R., R.S. Anderson, S.P. Anderson, and E.D. Waddington. "Numerical Simulations of Glacial-Valley Longitudinal Profile Evolution." Geology 28 (2000): 1031-1034. The Geological Society of America . Indiana University-Bloomington, Scottsdale , AZ. 26 Dec. 2007. Keyword: Glacier, Landforms, Yosemite .
Matthes, Francois E. Geologic History of the Yosemite Valley . United States Department of the Interior and United States Geological Survey. 1930. 24 Dec. 2007 <http://www.nps.gov/history/history/online_books/geology/publications/pp/160/contents.htm>.
Pinkers, Steven. " Yosemite Falls." Harvard University . 26 Dec. 2007 <http://pinker.wjh.harvard.edu/photos/american_west/pages/Yosemite%20Falls.htm>.
"Rivers of Ice." Kluane National Park . 21 Dec. 2007 <http://www.jovial.on.ca/vica/Arctic/Kluane/YukonG.jpg&imgrefurl>.
Schaffer, Jeffrey P. One Hundred Hikes in Yosemite: Evolution of the Yosemite Landscape-the Nevadan Orogeny . 5th ed. Wilderness P, 2004. Evolution of the Yosemite Landscape-the Nevadan Orogeny . 22 Dec. 2007 <http://gorp.away.com/gorp/publishers/wildernesspress/yosemite/hik_yosem25.htm>.
" Yosemite Falls Facts and Geology." Yosemite . Yosemite National Park . 26 Dec. 2007 <http://www.yosemite.ca.us/yosemitefallsfacts.html>.