ABSTRACT
To understand more about the earth's history, humans have often looked to the natural environment for insight into the past. The bristlecone pine ( Pinus longaeva ), of the White Mountains in California , has served this purpose greater than any other species of tree on the planet. With some living specimens attaining ages nearing 5,000 years, and dead matter persisting for another several thousand, these ancient trees have provided climatologists, geologists, archaeologists, and dendochronologists with a vital and continuous tree-ring chronology that dates back to the last Ice Age. In this regard, the bristlecone pine's contribution to science has been invaluable, forcing “a reevaluation of history itself” (Little, 2004). In addition to discussing the bristlecone's many contributions to science, this paper accumulates some basic knowledge of this species' complex physiology and habitat, drawing upon research from the past half century. This big picture is intended to illuminate the magnificence of bristlecone pines as living evidence of millennia gone by.
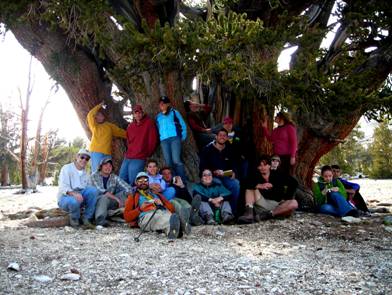
They are the old men of the forest, of the world—
gnarled, wizened, stripped of all the inessentials,
living longest in the heights, along the borders
with the iron sky, embracing cold. Their being,
granite hard, is dense enough to break a blade;
- Catharine Savage Brosman, 2006
::Introduction::
Photographed above are the members of Indiana University 's G190: Volcanoes of the Eastern Sierra Nevada . Together we embarked on a two-week journey through east-central California , examining several aspects of this region's natural history. In this illustration we gather in front of “the Patriarch,” the world's largest bristlecone pine. This particular tree is just one of thousands that grow atop California 's White Mountains, a high altitude range just east of the Sierra Nevada .
Conditions here are brutal: scant precipitation and low average temperatures mean a short growing season, only intensified by ferocious wind and mal-nutritious rocky soil (Gidwitz, 2001; The Old Man , 2002; Little, 2004). Nevertheless, bristlecone pines have claimed these barren slopes as their permanent home. Evolving here in this harsh environment, super-adapted and without much competition, bristlecones have earned their seat on the longevity throne by becoming the oldest living trees on the planet. Results of extensive studies on bristlecone pine stands have shown that in fact such, “environmental limitations are positively associated with the attainment of great age” (LaMarche, 1969, quoted from Schulman, 1954). This intriguing phenomenon will be discussed further on.
But exactly how old is old? Sprouted before the invention of Egyptian hieroglyphs and long before the teachings of Jesus of Nazareth, “Methuselah” (named appropriately after the long-lived biblical figure) is the oldest bristlecone alive at roughly 4,700 years. Although specimens of this age do not represent the species' average, “there are [at least] 200 trees more than 3,000 years old, and two dozen more than 4,000” (Gidwitz, 2001). Considering that these high ages are obtained in the face of such remarkable environmental adversity, the bristlecone pines have become the focus of much scientific examination over the past half-century.
Perhaps most interested in the bristlecone pine are dendochronologists, or tree-ring daters. With every strenuous year that passes in the White Mountains , each bristlecone grows and forms a new outer layer of cambium that reflects a season's particular ease or hardship. So while, “growing seasons may expand or shrink, the trees carry on, their growth rings faithfully recording the bad years alongside the good” (Hall, 2006). Through examining the annual growth rings of both living and dead specimens, taking thousands of core samples, and by processes of cross-dating between trees and other qualitative records, scientists have compiled a continuous tree-ring record that dates back to the last Ice Age between eight and ten thousand years ago (Feng and Epstein, 1994; Little, 2004). Among other linked accomplishments, this record has enhanced the dating process, helping to double-check and correct the radiocarbon-14 method to more accurately estimate the age of organic material.
Now more than ever the importance of monitoring the bristlecone is being realized. As our global climate continues to undergo its most recent and abrupt atmospheric change, these ancient scribes continue to respond. Since, “the rings of wood formed each year reveal the trees' response to climatic conditions during a particular growing season” (Simon, 1984), in their persistence they have left us natural recordings of the past, markers of the present, and clues to the future.
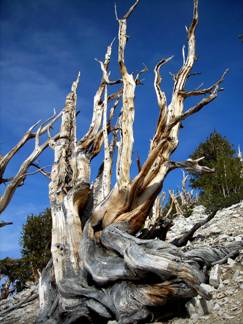
::Phenotype and Physiology::
Like all pines, bristlecones belong to the phylum Coniferophyta: the conifers. Classified within this phylum are almost fifty genera and roughly 550 species. It has been calculated that the Coniferophyta developed some 300 million years ago in the Carboniferous Period, branching into the pine family after another 165 million years of evolution. 135 million years later, this family presently includes bristlecones and coastal redwoods, respectively holding records for the oldest and tallest trees in the world (Allaby, 2001).
Although maintaining easily identifiable conifer traits, the bristlecone pine is certainly one of the oddest looking trees a botanist could imagine. Many have been crippled and gnarled over time, resulting in ghostly entangled forms of both living and dead material. Some researchers believe that in fact, “over the millennia bristlecones genetically programmed to twist may have been better adapted to survive” (Little, 2004), potentially explaining the unusual contortions. Deadwood colors range from white to dark gray or black; living wood ranges from light to dark brown. Because these trees grow for so long and at such slow rates, wood tends to be tightly compact, resinous, and highly resistant to disease, infestation, or rot ( Ferguson , 1968). For their old age bristlecones are also remarkably small, hardly ever exceeding thirteen meters tall by four meters around (Gidwitz, 2001).
The species' name originates from the appearance of its unusual cones and needles. Both male and female cones, generally five to eight centimeters long, have distinctively sharp bristles and exist on the same tree. To tell the difference, “the male cones are short and cylindrical, and are catkin-like. The female cones have a central axis bearing spirally arranged bract-scales, in the axils of which eventually arise the fertile scales” (Hora, 1981). The bristlecone's short, pale needles are also trademarks, bunching together to form foxtail-like bundles (Phillips, 1978). As is the case of most conifer needles, these specialized leaves cluster together to shelter the stomata so “very little moisture is lost through them” (Allaby, 2001). Needles on bristlecones are also exceptionally long-lived, each potentially functioning for up to forty years. This adaptation helps the bristlecone photosynthesize during particularly brutal months, “saving the energy of constant needle replacement and providing a stable supply of chlorophyll” (Little, 2004). Photosynthesis levels of these needles peak at temperatures between 10C and 15C (Mooney, West, and Brayton, 1966), and, “at least half of the summer growing season is needed to store enough carbohydrates to survive a normal winter. This result is certainly in agreement with the extremely slow growth of bristlecone pine” (Schulze, Mooney, and Dunn, 1967).
For a plant trying to store so much energy, bristlecone seeds are relatively large in size. They are first reproduced when trees reach ages between thirty and seventy-five years old. Germination rates are generally high, in part because seeds require little to no initial stratification (Hiebert and Hamrick, 1983). Although these seeds are winged, the wind is not their most prolific disperser. That title belongs to the Clark 's nutcracker ( Nucifraga columbiana) , a corvid family member with a powerful bill. Although the nutcracker prefers limber or pinyon pine seeds, bristlecone seeds are in greater abundance year-round (Lanner, 1988). Since a great percentage of the nutcracker's high-protein payload is stored away for later days, a surplus is gathered and cached. These birds are busy from August through December and a single nutcracker may store as many as 33,000 bristlecone seeds in a season. “This overstorage is significant, as seeds that aren't recovered can sprout into new pines, thereby benefitting both the trees and future generations of nutcrackers” (Friedcrici, 2000).
Perhaps the most intriguing physical characteristic of a mature bristlecone, however, is its ratio of living to dead wood on harsh sites and how this relates to old age. As saplings, tree trunks are straight, bark is healthily all-encompassing, and foliage crowns are dense (Connor and Lanner, 1990). In older trees, however, especially in individuals over 1,500 years, a “strip-bark” trait is adaptive. This condition occurs as a result of cambium dieback, which erodes and thereby exposes certain areas of the bole, leaving only narrow bands of bark intact (Beasley and Klemmedson, 1980). Factors leading to cambial area reduction include, “slow growth rates, wind damage, soil erosion…[and] repeated loss of foliage” (LaMarche, 1969). Also referred to by Schulman and Ferguson as “cambial edge retreat,” dieback is not detrimental to the plant, but rather quite helpful. As a result of this retreat, incomplete, arc-like ring segments are formed instead of whole ones, allowing the tree to, “maintain a constant ratio of green to non-green tissue almost indefinitely” (LaMarche, 1969). This adaptation will constantly preserve the most efficient photosynthetic level for the plant to survive.
The technique of cambial edge retreat has help promote old age in bristlecone pine, but that certainly is not the only reason. Most crucial to these trees' longevity is their compact size and slow rates of growth (Wright and Mooney, 1965). By remaining in most cases under ten meters tall, bristlecones stay close to the limited water supply and can hence support more branches and photosynthesizing leaves (Thomas, 2000). Combined with the dry, windy, and often freezing mountain air, slow growth guarantees the bristlecones tight, fibrous rings with a high resin content and structural strength. This resilience not only sustains the living trees but also preserves the dead, in some cases over an additional millennium (Gidwitz, 2001). In some extreme cases, “tree-ring and radiocarbon dating have shown that many logs and remnants found lying on the ground surface are from 4,000 to 7,000 years old, and one remnant has been found to be about 9,000 years old” (LaMarche and Mooney, 1972).
The absence of natural disaster has also safeguarded the bristlecone's lengthy lifespan. Due to a lack of ground cover vegetation and an evenly spaced layout, bristlecone stands on the White Mountain peaks (from 10,000 to 11,000 feet) have been practically unaffected by fire (LaMarche, 1969). This lack of vegetation also means a lack of competition for the bristlecones. “On sites with better growing conditions competition takes its toll of bristlecone pine, and longevity of trees is greatly reduced compared to harsher sites” (Beasley and Klemmedson, 1980). The simultaneous occurrence of the above-mentioned factors have led researchers to believe that, “environmental limitations are positively associated with the attainment of great age by bristlecone pines” (Schulman, 1954; LaMarche, 1969).
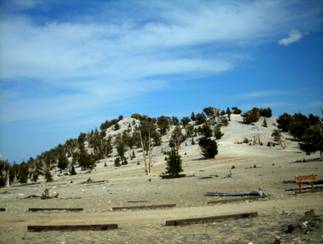
:: Natural Range and Growing Adversities::
“Bristlecone pine…is restricted to numerous, rather isolated stands at higher altitudes in the southwestern United States . Stands occur from the Rocky Mountains, through the Colorado Plateau, to the western margin of the Great Basin ” (Currey, 1965). Within this natural range, the oldest and most widely researched stands of bristlecones occur in California 's White Mountains . Even just 200 miles away from the Pacific Ocean, the White Mountains are home to one of this country's few high-elevation deserts. Located in the extreme eastern rain shadow of the Sierra Nevada ( Ferguson , 1968), this region receives only 12.54 inches of precipitation per year and experiences temperatures between -20F and +50F (Wright and Mooney, 1965; Feng and Epstein, 1994).
The peaks south of the Owens Valley , shown here, are higher up than they might appear from a distance. Although most summits exist somewhere around 11,000 feet, snow-capped White Mountain Peak , for which the range is named, stands at 14,246 feet above sea level (Wright and Mooney, 1965). That said, to reach areas of pure bristlecone is an intense journey all to itself. Our vans were forced to endure several thousand feet of windy dirt roads before our goal was even in sight: the Patriarch Grove. Part of the 28,000-acre bristlecone preservation effort in California 's INYO National Forest , this remote stand at 11,500 feet offers the public an insight into the bristlecone's glory (Little, 2004). Traveling there truly does make a student appreciate their seclusion and look a bit closer at their natural environment.
One of the first things many visitors to the bristlecone stands will notice, for instance, is the unusual soil composition. The characteristically thin, flat rocks are dolomite, a kind of limestone common to this sub-alpine region. Although sandstone, quartz, and granitic substrates are also present on the White Mountains ' upper elevations, it is the dolomite soils which promote the longest-lived bristlecones. This rock has weathered to form a sandy, alkaline loam that is rich in calcium and magnesium but low in phosphorous, clay, carbon, and nitrogen. Generally grayish-white in color, dolomitic rocks maintain lower temperatures than other substrates because of its high albedo. In this way, dolomite-derived soils can retain an optimum amount of precious moisture in the face of precipitation scarcity (Wright and Mooney, 1965; Beasley and Klemmedson, 1980).
With such a harsh, dry environment presented in this region, people often wonder how the bristlecone came to be there in the first place. Two explanations have been presented for their existence exclusively along the mountaintops. The first, which I have discussed already, involves a long-range dispersal of seeds by birds (nutcrackers) or potentially hoarding rodents. The other scenario, which is more thoroughly backed in the literature, suggests that the bristlecones originally covered the entire mountainside, “followed by the extinction of low altitude stands during the inter-glacials, leaving isolated mountain top populations” (Hiebert and Hamrick, 1983).
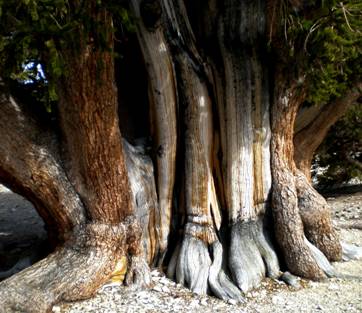
::Contributions to Science::
With seemingly endless areas of wonder and interest, the bristlecone pines have become subject to much research over the past half-century. Since the annual growth of these ancient organisms directly reflects the climatic conditions of a particular time period, bristlecones are of greatest significance to dendochronologists, or tree-ring specialists. Dating any tree is simple and can be done within reasonable accuracy just by counting out the rings made each year by the plant's natural means of growth. By carefully compiling a nearly 10,000-year-old bristlecone pine record (the longest derived from a single species), these patient scientists have accurately corrected the carbon-14 dating method and estimated ages of past periods of global climate change (Gidwitz, 2001). What makes this record so special to dendochronologists, too, is that, “nowhere, throughout time, is precisely the same long-term sequence of wide and narrow rings repeated, because year-to-year variations in climate are never exactly the same” ( Ferguson , 1968).
Completing this tree-ring record was no easy task. Considered one of the founding fathers of dendochronology, Edwin Schulman led much of this effort with a Swedish increment borer in hand. Throughout much of the 1950s, Schulman devoted himself the bristlecone, relentlessly searching for answers to their mysterious existence. He, like many others to come, saw their true potential as a scientific tool. Especially after his 1958 discovery of Methuselah (Gidwitz, 2001), Schulman drew attention to the bristlecones by publishing several of his findings. The first chronology he produced only reached back in time 3,000 years, but today, after many more years of core sample study and overlapping tree-ring patterns, the record now extends through the Little Ice Age, nearly 10,000 years. This record is now accessible to the climatological, archaeological, and geological greater good (Beasley and Klemmedson, 1980), as it has “enabled [all scientists] to recognize patterns in climate and environmental conditions” (Little, 2004) over an extensive period of time.
The 2002 edition of the American Heritage Student Science Dictionary defines dendochronology as, “The study of annual rings in trees in order to analyze past climate conditions or to determine the date of past events.” Since tree growth patterns innately respond to ecological stress, the width of a given year's tree-ring will correspond to that same year's level of environmental stress. Hence, a wide annual ring indicates a year of more favorable growing conditions, whereas a small or potentially non-existent ring signals one of adversity. Trends seen between these rings can tell dendochronologists a great deal. For example, as Beasley and Klemmedson (1980) describe, “Mean sensitivity, defined as the average ratio of the absolute difference in width between each two successive rings divided by the mean width of rings in the series, is an excellent index of the limiting effects of climate on growth rings.” Note that due to the bristlecone's slow growth rates, even a “wide” ring can be less than a hundredth of a millimeter across (Gidwitz, 2001; Ferguson, 1968). But by using technologically advanced microscopes, events of natural disturbance such as volcanic eruptions or glaciation (Currey, 1965) can still be observed within these tiny rings. After an explosive eruption for example, large amounts of pyroclastic material can fill the atmosphere and significantly drop regional temperatures as the dust particles block (reflect or absorb) incoming radiation. These sudden changes in climate can be seen as frost-rings, or, “hairline-thin zones of weakened cells” (Simon, 1984). Examining such areas of stunted growth in the bristlecone pine record has helped date several major eruptions worldwide, including Etna on Sicily and Santorini near the Aegean Sea – years 44 B.C. and 1627 B.C. respectively (Simon, 1984).
Although dating these disturbance events has been an important use of the bristlecone record, perhaps the most influential contribution has been correcting the previously inaccurate radiocarbon-14 dating method. This approach is made possible by incoming solar radiation that converts our planet's nitrogen-rich air into carbon-14. Joining with oxygen, this radioactive isotope morphs into carbon dioxide and is absorbed by plant respiration and the natural food chain. After an organism dies, the carbon isotope begins to decompose at a steady half-life rate of every 5,730 years. Then, to obtain the age of a specimen, scientists compare the amount of carbon-14 in it to the amount of carbon-12. By using this ratio and carbon- 14's half-life, they can determine the date the organism died” (Gidwitz, 2001). This approach was thought for years to be an accurate measure of time, but the dating of bristlecone pines with known ages brought up startling discrepancies. Now presented with a way to cross-check the carbon-14 technique, scientists compared the two records for calibration. Results showed that, “for material in the period 4,000 to 3,000 B.C., dates obtained by the conventional carbon-14 method are about 800 years more recent than dates established dendochronologically” ( Ferguson , 1968). This new discovery implied that many age estimates provided by carbon dating in the past were wrong, and could now be adjusted appropriately thanks to the ancient bristlecone pine (Little, 2004).
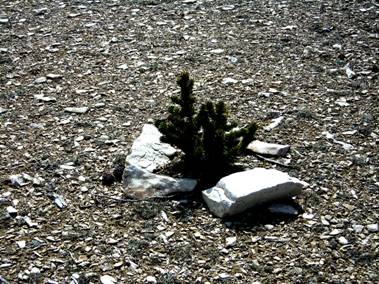
::Conclusion::
As our group left the Patriarch Grove that day, many appeared humbled by their bristlecone experience. One twenty-five-year-old sapling in particular, shown here above at only thirty centimeters tall, gave us an awe-inspiring perspective on these incredible plants. With a 5,000 year lifespan potentially ahead of it, this young tree symbolized the hope for all bristlecone – that their monumental existence will be preserved. Although increased awareness of the bristlecone has been beneficial to science, publicity may ultimately disturb these stands.
Historically the bristlecone's remote location and gnarled wood have deterred commercial extraction, but nothing on earth will go unaffected by global warming. “If temperatures rise by only 6 degrees F, which many experts say is likely this century, about two-thirds of the bristlecones' ideal habitat in the White Mountains effectively will be gone” (Hall, 2006). Almost 30,000 acres of National Forest now preserves the ancient bristlecone, but paved roads, campsites, and self-guided trails have led only to more human impact. In 1966, the U.S.F.S reported over 20,000 visitors to the Ancient Bristlecone Pine Forest, a figure which could exceed 40,000 today ( Ferguson , 1968). For these magnificent pines to truly be preserved, there must be a ubiquitous understanding of their significance. Over the past hundreds of thousands of years, this species has endured in one of earth's most trying environments; they deserve our respect and reverence. As global climate change slowly alters their environment, we as humans must do our part to raise awareness and lower our impact. This especially means a limitation of on-site collecting, which has become a greater problem since the roads' development. To conclude, C.W. Ferguson implores in his 1968 report, Bristlecone Pine: Science and Esthetics , “the need for protection may be lessened by such education of the visitor, which might lead him to appreciate the beauties of nature in terms of the interests of posterity rather than his immediate personal gain” ( Ferguson , 1968).
Work Cited
Thomas, Peter A, A Tree's Old Age. Natural History; May2000, Vol. 109 Issue 4, p96, 1p, 1bw
den·dro·chro·nol·o·gy, American Heritage Student Science Dictionary; 2002, p95-95, 1/6p, Reference Entry
Carl Hall Performing high-altitude research on global warming Environment, San Francisco Chronicle (CA), 08/02/2006
Simon, Cheryl, Frost rings used to date eruptions. Science News; 1/28/84 , Vol. 125 Issue 4, p54-54, 1/3p
Sun's future not so bright, Astronomy; Oct95, Vol. 23 Issue 10, p24, 1/4p, Publishing Co.
Gidwitz, Tom,Telling Time. Archaeology; Mar/Apr2001, Vol. 54 Issue 2, p36, 6p, 5c
Friedcrici, Peter, The Bird That Never Forgets, National Wildlife; Oct/Nov2000, Vol. 38 Issue 6, p32, 4p, 2c
THE OLD MAN, New Scientist; 10/19/2002 , Vol. 176 Issue 2365, p59, 1/2p, 1bw Reed Business Information UK, Ltd.
Little, Jane Braxton, Time Line. American Forests ; Winter2004, Vol. 109 Issue 4, p22-27, 6p, 3c
Wright, R.D. and Mooney, H.A., Substrate-oriented Distribution of Bristlecone Pine in the White Mountains of California.1965
Connor, Kristina F. and Lanner, Ronald M., Effects of Tree Age on Secondary Xylem and Phloem Anatomy in Stems of Great Basin Bristlecone Pine (Pinus longaeva) 1990
Beasley, R.S., and Klemmedson, J.O., Ecological Relationships of Bristlecone Pine 1980
LaMarche, Valmore C. Jr. and Mooney, Harold A., Recent Climatic Change and Development of the Bristlecone Pine (P. longaeva Bailey) Krummholz Zone, Mt. Washington , Nevada . 1972
Lanner, Ronald M., Dependence of Great Basin Bristlecone Pine on Clark 's Nutcracker for regeneration at High Elevation.1988
Mooney, H.A., West, Marda, and Brayton, Robert, Field Measurments of the Metabolic Responses of Bristlecone Pine and Big Sagebrush in the White Mountains of California. 1966
Connor, Kristina F. and Lanner, Ronald M. Cuticle Thickness and Chlorophyll Content in Bristlecone Pine Needle of Various Ages. 1991
Billings , W.D. and Thompson, J.H., Composition of a Stand of Old Bristlecone Pines in the White Mountains of California . 1957
Currey, Donald R., An Ancient Bristlecone Pine Stand in Eastern Nevada . 1965
LaMarche, Valmore C. Jr., Environment in Relation to Age of Bristlecone Pines. 1969
Schulze, Mooney, and Dunn, Wintertime Photosynthesis of Bristlecone Pine (Pinus Aristata) In the White Mountains of California . 1967
Hiebert, Ronald D. and Hamrick, J.L. Patterns and Levels of Genetic Variation in Great Basin Bristlecone Pine. 1983
Adam, David P., Ferguson , C.W., and LaMarch, Valmore C. Jr., Enclosed Bark as a Pollen Trap. 1967
Feng, Xiahong and Epstein, Samuel, Climate Implications of an 8000-Year Hydrogen Isotope Time Series from Bristlecone Pine Trees. 1994
Ferguson , C.W., Bristlecone Pine: Science and Esthetics. 1968
Torick, Lisa L., Tomaback, Diana F., and Espinoza, Ronald, Occurrence of Multi-genet Tree Clusters in “Wind-dispersed” Pines
Phillips, Roger, Trees of North America and Europe . 1978 p 152. Random House, NY.
Hora, Bayard, The Oxford Encyclopedia of Trees of the World. 1981. Oxford Press, NY
Allaby, Michael, Plants and Plant Life. 2001 – vol 8: Conifers – Grolier pub
http://sonic.net/bristlecone/
http://gorp.away.com/gorp/resource/us_national_forest/ca/see_iny1.htm
http://www.pbs.org/wgbh/nova/methuselah/long.html
http://www.birds.cornell.edu/AllAboutBirds/BirdGuide/Clarks_Nutcracker.html
http://web.utk.edu/~grissino/principles.htm#3 Grissino-Mayer, Dr. Henri D., Ultimate Tree-ring Web Page - Principles of Dendrochronology. University of Tennessee , 2007
Image Credits:
*all photos by either Amanda Brooke Leavitt or Patrick Woodson.