ABSTRACT
This paper explores the particular geological circumstances that give rise to hydrothermal systems. In certain areas, groundwater is circulated deep beneath the Earth’s crust to be heated by magma, only to rise to the surface once more and manifest itself in the form of hot springs and fumaroles, leaving underground mineral deposits in its wake. An emphasis is put upon such phenomena along mid-ocean ridges, their peculiar ecosystems and chemistry, and particularly hydrothermal circulation within the Sierra Nevada Range, where hydrothermal systems are exploited in order to generate geothermal power, prospect for mineral veins, and detect impending earthquakes and volcanic eruptions.
I. Introduction
The word “volcanism” is full of associations—volcanoes themselves are elemental forces that continue to scar continents and raise new land from the depths of the ocean. They capture the imagination with their immense size, violence, and seemingly unpredictable behavior. Yet much of the continental United States appears superficially far removed from any threat of eruption, at least within the scope of a human lifetime; to view a major volcanic eruption would be a singular occurrence, to be sure. However, a host of other volcanic phenomena are far more common and in some respects just as relevant to human civilizations. As magma rises within the Earth's crust, it often interacts with the local water table to produce hydrothermal systems, areas of heated groundwater that begin to cycle through the region and alter the chemistry of local rock formations as well as manifest themselves on the surface as geysers, fumaroles, and hot springs .
Hydrothermal circulation assumes importance for a variety of reasons. For instance, it can represent a significant economic boon to a region if properly exploited: Geothermal power can provide a relatively clean source of energy that can be derived from superheated water pumped from deep underground. Hydrothermal ore deposits can also lead to rich mineral veins as magma-heated waters cool and cause the dissolved minerals contained within them to precipitate near the surface. There are also environmental reasons for studying and monitoring these formations. The water level and output of hot springs and other hydrothermal features may vary considerably in response to seismic activity, such as earthquakes, or an impending volcanic eruption which could provide an early warning for local residents to evacuate their homes in anticipation of the impending tremor or lava flow.
Consequently, this paper will first examine the ways in which hydrothermal circulation can take place and the geological conditions that fuel it, followed by an overview of the many features that derive from these processes. Hydrothermal systems exist not only on land, but also on the ocean floor where they give rise to varied and unique ecosystems that thrive even in the absence of sunlight. A particular emphasis will be placed on the occurrence of hot springs and fumaroles in the Eastern Sierra Nevada range. This will be followed by a look at the economic uses of hydrothermal circulation for geothermal power and mining ore deposits, as well as earthquake and volcanic monitoring in the region.
II. Hydrothermal Physics and Chemistry
Hydrothermal systems that result in observable features such as hot springs are widely distributed throughout the globe, but are typically clustered together in areas with large amounts of preexisting volcanism. By proxy, this also associates them with tectonic plate boundaries and often regions of either mountain building via subduction of the ocean floor beneath a continental plate, or rifting zones where plates are spreading apart. The latter is particularly true of the Atlantic and Pacific Oceans , where hydrothermal vents are common along mid-ocean ridges. (Ellis and Mahon , 29) Additionally, sustained hydrothermal flows require a steady supply of water and an area of porous rock to allow that water to circulate deep enough underground to encounter magma or a layer of heated rock, which acts as a primary heat source. (Ellis and Mahon , 33)
There are two main ways in which this circulation of water can take place. The first involves what is known as “meteoric” water, or groundwater that has recently been in the atmosphere. As it begins to seep to a lower depth through fissures and cracks in the local bedrock, the meteoric water will ultimately begin to rise in temperature as it comes across either cooling magma or solid rocks themselves being heated from beneath by magma. As the water temperature rises, it becomes less dense and via convection will begin to move once more to the surface, retaining its heat and often bubbling out of a hot spring many miles away from where it originated, provided that the local rock is permeable enough to allow it to pass through. It is currently thought that most hydrothermal systems are supplied by meteoric water in this manner. (ODEX)
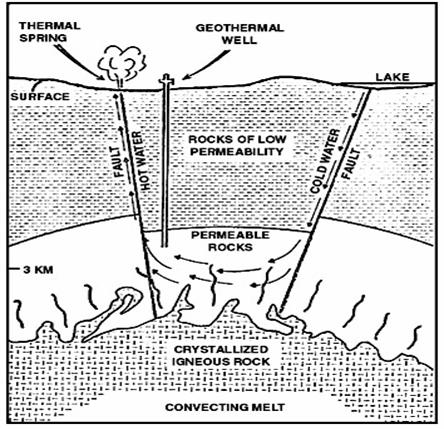
Fig. 1: Hydrothermal Circulation of Meteoric Water
Alternately, it is possible for water to originate at a low depth and later reach the surface. As magma cools, it slowly stratifies and releases small amounts of “magmatic” water, or water that has not been circulated through the atmosphere in recent geological times. This solution is typically rich in various dissolved ores, such as sulfur, iron, copper, or even gold. Similar to meteoric water, convection will cause this mineral-laden water to rise through cracks in the rock, possibly to reach the surface through a spring or geyser. In doing so, the magmatic water will deposit large veins of minerals in its wake. (ODEX)
It is often a difficult task in a field setting to determine which of these two methods or what combination of them feeds a particular hydrothermal field. While meteoric waters are thought to be the most prolific source overall, it is thought that considerable mixing of different types and temperatures of water may occur deep inside the Earth's crust, making it difficult to develop an accurate picture of how these processes in fact work. (Ellis and Mahon , 79) One method that has proved reasonably accurate involves measuring levels of tritium in water as it reaches the surface. Tritium is a radioactive hydrogen isotope with a half-life of 12.5 years, and is common in all atmospheric water vapor due to bombardment by interstellar rays. Thus, careful measurements of the tritium levels in the waters of hot springs can reveal how long they have been underground and thus removed from the hydrosphere. (Ellis and Mahon , 79) Circulation times of meteoric waters vary considerably, with measurements ranging from 50 to 30,000 years for an individual molecule to return to the surface once it has been drawn into a hydrothermal system. Large numbers of this kind may result from mixing with magmatic water, but there is no clear way of knowing for certain.
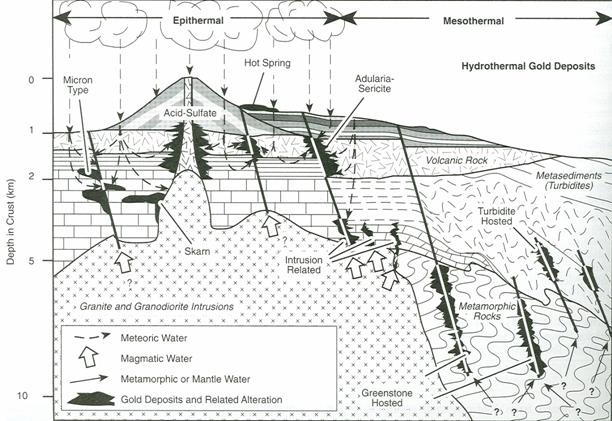
Fig. 2: Combined Hydrothermal Circulation of Meteoric and Magmatic Water
Numbers of this kind assume greater importance when discerning the origin of the various minerals found in hydrothermal waters. Large amounts of elements such as “lithium, rubidium, cesium, boron, fluoride, arsenic, carbon dioxide, and hydrogen sulfide” (Ellis and Mahon , 80) in water samples were originally considered ironclad evidence of a magmatic water source. However, the isotopic ages of water samples determined from tritium analyses cast doubt on that hypothesis, by demonstrating the young ages of certain samples. One alternative involves circulating meteoric water actually coming into contact with magma in the deep crust, passing through its upper layers and being forcibly mixed with dissolved minerals and magmatic waters before convection draws it to the surface once more. It is also possible that instead of directly interacting with magma, that meteoric water could draw minerals straight from superheated rocks deep in the Earth's crust, though this would vary greatly in composition from place to place. While it is difficult to prove either of these theories correct, they are by no means exclusive to one another, and it is probably that different combinations of the two work together to determine the mineral composition of hydrothermal systems in different parts of the world. (Ellis and Mahon , 80-81)
III. Deep Sea Hydrothermal Systems
The basic functioning of oceanic hydrothermal vents is similar to that which has already been discussed, but their ecological role is a unique and fascinating look at how animal species can adapt and survive in a near-alien environment. Hydrothermal vents are common in both the Atlantic and Pacific Oceans , forming primarily along mid-ocean ridges where magma is very close to the crustal surface. There is little doubt about the origin of the water involved in these hydrothermal processes: seawater seeps through the loose sediments and rifts in the ocean floor, encountering magma and heated rock hundreds of meters below. The temperatures at this depth may peak at as much as 200 degrees Celsius before the water once more convects upward carrying dissolved minerals such as methane, carbon dioxide, and various sulfides. (Desbruyères, 211)
In a process similar to the terrestrial formation of mineral veins, many of the minerals precipitate at once when they encounter cold seawater. Cascading upward from the ocean floor in black plumes of minerals, many of these vents coalesce into chimney-like structures called “black smokers,” which may rapidly grow as tall as 15 meters at a rate of 30 cm each day. (Tivey) In the chimneys' early growth period, the hydrothermal solution bursts out of the seabed at a velocity of 5 m/s at an initial temperature spike of roughly 350º C. Out of the mineral-rich water precipitates anhydrite, a compound formed from calcium dissolved in the hydrothermal solution and the sulfates naturally occurring in seawater. The anhydrite is soon reinforced by dissolved iron, copper, and manganese that make up the clouds of material giving black smokers their name, floating upward as high as 200 m above the chimney itself due to the hydrothermal water's high temperature and low density. (Tivey) These particles occasionally become lodged within the foundation of the anhydrite and slowly blacken the white calcium sulfate, as copper-iron sulfide begins to coalesce inside the formation's brittle walls, thickening them and defining the shape of the chimney. (Tivey) Mining these regions would be a very profitable business, were it not for the extreme difficulty in reaching the depth at which black smokers proliferate. Still, the water surrounding these vents is often 20º C warmer than the rest of the ocean floor, which hovers at a mere 2º C, providing a survivable habitat for numerous species that could not otherwise live in the bleak expanse of the deep sea. (Desbruyères, 211)
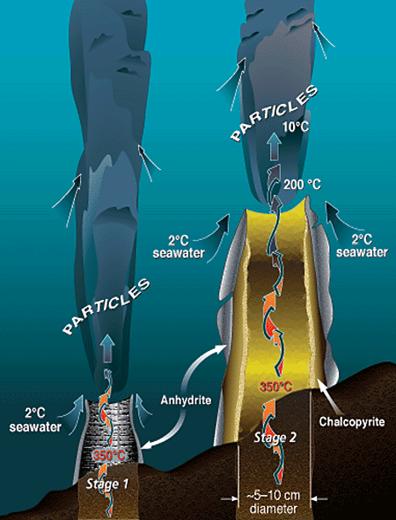
Fig. 3: Formation of a Black Smoker
This is not to say that these hydrothermal vents are inviting habitats, at least in a traditional sense. At a depth of 3000 meters, there is no natural sunlight to power photosynthesis for plants growth, in addition to the toxic mineral levels that seep out into the crushing depths of the ocean. The building blocks for such an ecosystem are very different than any other environment on Earth, since plant matter and thus the Sun cannot form the basis of a food chain. Rather, it is bacteria that makes life around a black smoker possible via chemosynthesis—the oxidation of hydrogen sulfide into usable nutrients. Rising in abundance from the hydrothermal vent, certain microorganisms are able to thrive and grow in boiling water—over 113º C—which is the highest temperature at which living organisms are known to survive and at a pressure that is 200 times that found on land. (WWF)
Various tubeworms, shrimp, crabs, and other crustaceans feed upon these bacteria and are able to sustain themselves in a manner that has likely remained unchanged for millions of years. Some scientists speculate that the first organic life arose in a hostile environment such as a hydrothermal vent, long before oxygen was dominant in Earth's atmosphere and any multicellular life had evolved. (WWF) Further scientific study is now underway to determine whether or not bacteria from these regions can be used in an industrial setting to break down various waste compounds such as hydrogen sulfide which today present a challenge to companies who wish to properly dispose of their own excess materials. However, it is important to maintain the integrity of these hydrothermal ecosystems as commercial and scientific interests converge upon their delicate habitats; the governments of both Canada and the Azores are the first to officially protect marine hydrothermal environments against future exploitation in an effort to preserve them against accidental damage, chemical imbalance, and the introduction of foreign species. (WWF)
IV. The Role of Hydrothermal Systems in the Sierra Nevada Range
Given the geological setting of the Sierra Nevada Mountains , it is no surprise that there is a rich network of hydrothermal activity in and around them. The mountains are near to the San Andreas Fault, where the Pacific and North American plates grind past one another, as well as the Great Basin, a large rift forming in the Western United States . As a consequence, the Earth's crust is extremely thin and there is a large amount of cooling magma available directly below the crust's surface to heat groundwater and power hydrothermal circulation.
California is in a good position to exploit many of the economic benefits of its hydrothermal activity. The state has a long and illustrious history in connection to mining rich and valuable ores left by hydrothermal deposits, and has already constructed a stable geothermal power plant at Casa Diablo, a field littered with bubbling hot springs and fumaroles. A fumarole is a thermal vent where a layer of magma or heated rock releases volcanic gas or steam due to more intense heat or a shallower depth when compared to the source of a hot spring.
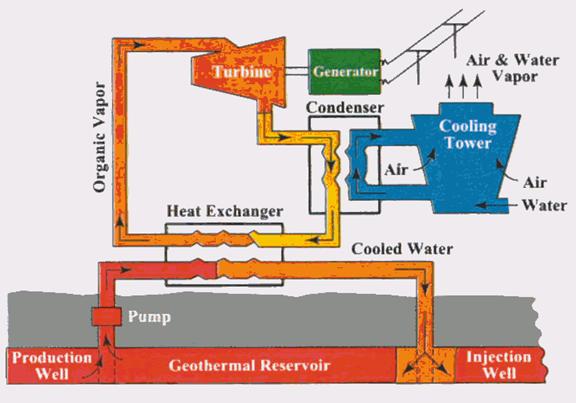
Fig. 4: Binary Cycle Power Plant
The Casa Diablo power plant takes advantage of the high concentration of fumaroles and springs in the area to drill deep wells from which superheated water can be pumped under pressure from depths between 300 and 2000 M below the surface. This water is often well above its usual boiling point, often as much as 150° C, but the intense pressure of the deep crust is enough to force it to remain in liquid form. (WEC) Upon reaching the surface, the water is used differently at different types of power plants. At Casa Diablo, this heated solution is used to evaporate large tanks of isobutene within a heat exchanger which in turn power turbines connected to an electrical generator, while other plants allow it to depressurize and “flash,” as it instantly turns to hot steam that rises and spins turbines directly. (WEC) In the case of Casa Diablo, the heated water is later recirculated back underground via a second deep well, and the isobutene is condensed as well for future use. The only significant amount of waste from the entire process is a layer of minerals that eventually cakes the water pipes, carried up and precipitated out of the water itself from the deep ground. This must be disposed of yearly, and stored in a hazardous waste facility. However, the facility is able to sell an average of 9 megawatts of energy at any given time, as well as provide their own power to keep the plant operational. Admittedly, this is a small amount compared to a coal-burning power plant, but nonetheless it is a step towards renewable power. (WEC)
In this way, geothermal power has the potential to become an important part of our daily lives, since it represents a clean if expensive means for generating electricity. However, it used as a city's sole source of power, several flaws become apparent with the use of geothermal plants. In the first place, they are highly expensive to install, requiring a great deal of capital investment before any returns can be made. Secondly, in an area as seismic as the Sierra Nevada , there is a question of reliability: a tremor or major earthquake could potentially damage or even destroy most of the hydrothermal systems in the area by simply blocking the artificial wells and natural pathways followed by the water through the bedrock, adding additional risk to the venture. Furthermore, while hydrothermal systems of this kind are ostensibly renewable, their natural rate of recharge is less than the amount of water that would be withdrawn from them through artificial wells if they were exploited at their maximum industrial capacity. Thus, while there would be ample heat remaining below ground, it would be necessary to halt energy production in order to let the water regenerate itself for an extended period of time. (WEC) Thus, while geothermal power is an attractive method for generating large amounts of power in an environmentally-friendly manner, it cannot assume the sole energy burden now put upon fossil fuels.
In addition to use for fulfilling its power requirements, research is now being done in the Sierra Nevada upon geysers for predicting earthquakes, a potentially major issue in the western state. Though relatively common and typically sedate, a single massive earthquake could devastate a large part of a city such as Mammoth or Los Angeles and result in many deaths as a worst case scenario. The phenomenon that is currently under scrutiny is the measurable change in the period between eruptions of geysers that adhere to regular schedules. Typically, following seismic activity, the period between eruptions is know to increase in geysers, as was observed at Old Faithful in Yellowstone National Park during the early 1980s. The earthquake in question took place at Borah Peak some 240 kilometers away from the geyser, measuring a 7.3 on the Richter scale. (Silver and Valette-Silver, 1364) However, it is suggested that in the days preceding such a large earthquake, similar changes can be observed in the eruption patterns that could be used to predict the disaster.
A geyser functions very much like a typical hot spring, except that as hot water rises to the surface, it encounters cold water that slowly heats upon contact with the hydrothermal solution from the deep ground. As this occurs, the cold water continues to exert large amounts of pressure that keeps the boiling hot water in liquid form, until at last the channel leading to the surface is filled with boiling water and erupts in a cloud of steam and scalding liquid. High-silica rocks such as rhyolite lava flows have left behind are particularly well suited to this sort of activity. (Ellis and Mahon , 37)
Ultimately, monitoring of Calistoga Geyser in the Sierra Nevada Range in 1992 uncovered several correlations between the geyser's eruption pattern and regional earthquakes. Following a major earthquake at Loma Prieta, the eruptions of the Calistoga Geyser came at 120 minute intervals for roughly two months, up from the 90 minute intervals that were standard beforehand. However, close examination of the recorded eruption data showed that this change in the eruption period actually began 60 hours prior to the earthquake itself. Similar trends became apparent for other earthquakes in following years, and barring other influences such as changes in precipitation or barometric pressure, it seems likely that at least in certain cases it might be possible to predict Californian earthquakes in the short-term. (Silver and Valette-Silver, 1366)
A paradigm explaining this phenomenon has not yet come to light, yet three are some indications as to how the geysers may be related to distant earthquakes. That itself is part of the issue at hand—Calistoga Geyser reacted to a large earthquake at a distance of over 150 kilometers. It is possible that the early stages of the earthquake were responsible for diverting water away from the geyser in some way, altering its flow rate, though it is still unclear how this process works. (Silver and Valette-Silver, 1367) Nevertheless, it is not unreasonable to suppose that in time a better understanding of geysers and earthquakes will allow for accurate predictions before they strike California .
V. Conclusions
Hydrothermal systems are certainly relevant to geological studies, but as time goes on they begin to assume a greater importance with regards to society, as human needs for new power sources and sources of raw materials grow and require immediate solutions. Regions rich in such resources, such as the Sierra Nevada, will likely exploit them but must remains alert to the fact that the internal workings of these systems are delicately balanced and not receptive to tampering—circumspect management of these valuable resources will keep them in such a condition that they will outlast the industrial need for them, and remain available for all to view and study in the distant future.
Works Cited:
Desbruyères, Daniel. “Hydrothermal Vents.” The Deep . Ed. Claire Nouvian. Chicago : University of Chicago Press. 2007. 211-224
Ellis, A.J., and W.A.J. Mahon. Chemistry and Geothermal Systems . New York : Academic Press, 1977.
Silver, Paul G., and Nathalie J. Valette-Silver. “Detection of Hydrothermal Precursors to Large Northern California Earthquakes.” Science . 257.5075 (1992): 1363-1368
Stanford Ore Deposits EXploration research group (“ODEX”). August 5, 2000: http://pangea.stanford.edu/research/ODEX/group.html Accessed June 13, 2007
Tivey, Margaret Kingston. “How to Build a Black Smoker Chimney: The formation of mineral deposits at mid-ocean ridges .” December 1, 1998: http://www.whoi.edu/oceanus/viewArticle.do?id=2400 Accessed June 13, 2007
World Energy Council. (WEC) “Survey of Energy Resources: Geothermal Energy.” http://www.worldenergy.org/wec-geis/publications/reports/ser/geo/geo.asp Accessed June 13, 2007
World Wildlife Fund. (WWF) “ Oases on the ocean floor.” June 19, 2002: http://www.panda.org/about_wwf/what_we_do/marine/news/stories/index.cfm?uNewsID=2593 Accessed June 13, 2007
Image Credits:
Figure 1: http://www.gi.alaska.edu/ScienceForum/ASF0/087.html R. B. Forbes and N. Gibbar , Northern Engineer , 5 Spring, 1973. Accessed June 13, 2007
Figure 2: http://serc.carleton.edu/research_education/nativelands/ftbelknap/golddeposits.html Kesler, S.E., 1994, Mineral Resources, Economics and the Environment : Macmillan , New York , 394 p. Accessed June 13, 2007
Figure 3: http://www.whoi.edu/oceanus/viewArticle.do?id=2400 Tivey, Margaret Kingston. “How to Build a Black Smoker Chimney: The formation of mineral deposits at mid-ocean ridges .” December 1, 1998. Accessed June 13, 2007
Figure 4: http://www.worldenergy.org/wec-geis/publications/reports/ser/geo/geo.asp Geothermal Energy , 1998, University of Utah Accessed June 13, 2007