Abstract
Geothermal energy – natural heat from the earth's interior – offers a clean and sustainable alternative to fossil fuels. Geologic processes such as plate tectonics and volcanism allow this energy to be concentrated in the upper portion of the earth's crust, and combined with hydrothermal systems, provide the means for this energy source to be utilized. Geothermal fluids of varying temperatures are used in direct heating applications and for the production of electricity with minimal environmental impacts, though certain environmental issues should be considered when developing geothermal resources. As technologies continue to improve and the demand for sustainable energy goes up, geothermal energy can play a major role in solving the global energy problem.
Introduction
Geothermal energy is a clean and abundant energy source that offers a sustainable alternative to fossil fuels, and which exists right under our feet! Geothermal energy has been harnessed for a variety of purposes in countries around the world, but this vast energy resource is largely untapped.
The value of geothermal energy has been known for years, since the first geothermal power plant went into production in the early 20 th century. It wasn't until the oil crisis of the 1970s, however, that the Federal Government accelerated the development of geothermal energy on a large scale [Duffield and Sass, 2005].
The goal of this paper is to explain the basis of geothermal energy, how it is utilized in a variety of different applications, and the different environmental concerns associated with the exploitation of these geothermal resources. The development of geothermal energy as a major energy source in the world will also be highlighted.
Natural Energy From the Earth
The word geothermal refers to the heat of the Earth's interior, and is derived from the Greek words geo (meaning Earth) and therme (meaning heat) [http://geothermal.marin.org/] . The intense heat within the Earth's core is partly a remnant of its fiery formation, but the decay of radioactive isotopes also supplies a steady source of heat within the Earth [Hulen and Wright, 2001].
The amount of energy inside the Earth is incredibly vast. Keller [2000] relates that 1 percent of the total energy in the upper 10 kilometers of the crust is equal to 500 times the global oil and gas resource. The critical problem is how all this energy can be developed and effectively turned into useful power.
In certain regions, geological processes – mainly, those of plate tectonics and volcanism – have caused bodies of molten and hot igneous rock to intrude into shallow depths of the crust. In these areas, enormous amounts of heat are concentrated near the surface, thus creating a steep geothermal gradient [Keller, 2000].
In Environmental Geology , the geothermal gradient is explained as the increase in temperature with depth below the earth's surface, measured in degrees per kilometer; the steeper the gradient, the greater the heat flow to the surface [Keller, 2000]. While volcanic regions have a steeper geothermal gradient (temperatures in excess of 350 ° C are found as little as 1 km below the surface), most regions of the Earth have a “normal” geothermal gradient [Mock et al., 1997].
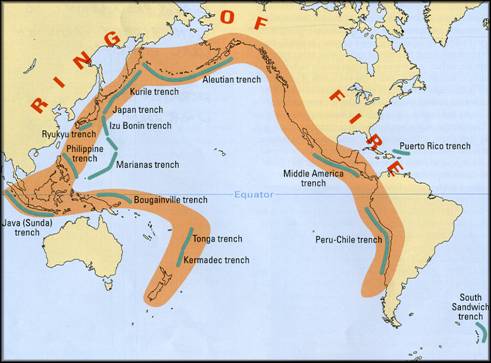
Image showing areas ripe for geothermal development. Source: USGS Circular No. 1249
In the Midwestern United States, temperatures hot enough to be useful as a geothermal resource are located at depths too far below the surface. For this reason, Duffield and Sass [2005] suggest that prime target areas for the development of geothermal energy resources are plate-boundary zones and regions affected by recent volcanic activity, as demonstrated in the preceding illustration.
Hydrothermal Resources
It is important to understand that the most valuable (and most exploited) geothermal resources would not exist without the occurrence of natural hydrothermal-convection systems, which facilitate the transfer of heat energy to the surface.
Hydrothermal environments are created as groundwater (which flows underground through fault structures and porous rock) interacts with a heat source. The permeable zone of water accumulation is typically contained within a region of less permeable rock or sediments [Keller, 2000], creating a reservoir of highly pressurized, high-temperature geothermal fluids.
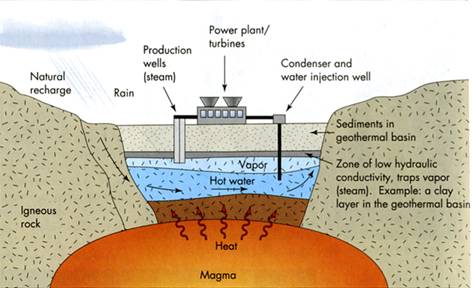
Illustration shows idealized geothermal resource. Source: Environmental Geology, Prentice Hall, 2000.
When released out of the ground, these geothermal fluids (or brines, i.e. highly saline water, rich in gases and minerals) create the surface manifestations typically associated with hydrothermal systems. Geysers, boiling mud pots, hot springs, and fumaroles (or hissing steam vents) are all features which have long been appreciated for their inherent scenic and recreational values [Duffield and Sass, 2005].
Geothermal resources that rely on hydrothermal systems are typically divided into categories based on heat content. Low- to moderate-temperature resources are often used for recreational and direct-heating purposes in a variety of settings. Moderate- to high-temperature resources are used not only used in electric power production, but also for direct-heating purposes in industrial settings.
Direct-Use of Geothermal Energy
Geothermal energy has long been used for bathing, heating, and cooking since the beginning of recorded history. Low-temperature systems such as hot springs were used by the ancient Japanese, Greeks, and Romans, who enjoyed the recreational and therapeutic values of geothermally-heated spas and bath houses [Duffield and Sass, 2005], but these geothermal resources were also used to heat buildings in ancient times.
There are a variety of commercial and industrial applications that utilize direct-heat from low- to moderate-temperature geothermal systems (ranging in temperatures from 21 ° C to 149 ° C). Applications include health spas and resorts, fish hatcheries, and greenhouses (for the growing of fruits, vegetables, and flowers) [Hulen and Wright, 2001].
Geothermal resources have been valued for direct-heating purposes in the United States since 1892, where geothermal fluids heated buildings in Boise, Idaho [Hulen and Wright, 2001]. To date, 18 different communities in the western United States use geothermal heat systems to heat homes, businesses, and civic buildings [Duffield and Sass, 2005].
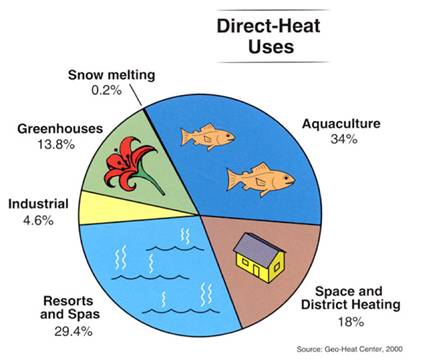
This pie chart illustrations the most common direct uses of geothermal energy.
Electricity From Geothermal Energy
The first geothermal power plant was developed in Lardarello, Italy in 1904, where natural steam from the earth was used to drive a turbine-powered electric generator. This experimental method of tapping the Earth's energy via hydrothermal systems caught on, and by 1960, the first geothermal power plant in the United States opened in The Geysers field in California.
The three systems used to generate electricity from geothermal energy are vapor-dominated, flash-steam, and binary systems (illustrated in the following image). Duffield et al. [2005], explains the basis of these systems, which are related in the following descriptions.
Vapor-Dominated Systems
The rarest and most valuable hydrothermal resources are vapor-dominated systems, which have been exploited by geothermal power plants such as those in Lardarello and The Geysers field. In vapor-dominated systems, reservoirs of highly-pressurized steam (at temperatures in excess of 235 ° C) are contained in porous and permeable rock settings. Th is steam is extracted through production wells and used to spin turbines which generate electricity. Condensers positioned after the turbine collect wastewater, which is injected back into the steam reservoir. Returning geothermal fluids to their source reservoirs at the rate they are extracted helps to maintain pressure within the hydrothermal system, and ensures that the resource remains productive and useful.
Hot Water Systems
Hot water systems (also known as flash-steam systems) use production wells to transfer highly pressurized water of about 200 ° C to the surface from as deep as 4 km underground. When the water rises up through the wells, 30% to 40% flashes to steam as the pressure drops closer to the surface. This steam is then routed to turbine units which generate electricity; unused water is injected back into the reservoir to maintain the productivity of the plant.
Binary Systems
The Mammoth-Pacific geothermal plant outside of Mammoth Lakes, California, provides a good example of a binary system being used to generate electricity. In this system, geothermal fluids of about 170 ° C in temperature are pumped to the surface and kept under pressure to prevent them from boiling over. They are then piped to heat exchangers which transfer their heat to a working fluid (in this case, isobutane), which has a lower boiling point than water. The isobutane vaporizes, drives a turbine power generator, and then returns to a liquid state, again ready for use; the geothermal fluids (after heat transfer) are returned to the subsurface reservoir through injection wells. This type of system is considered closed-loop, since all of the fluids and gases are contained within the system.
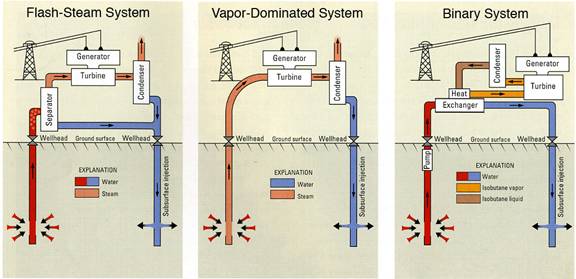
Source: USGS Circular No. 1249
Environmental Impacts of Geothermal Development
It is largely believed that geothermal energy resources allow for the clean generation of electricity with the least environmental impact. This is true to an extent – compared to fossil-fueled plants, geothermal power plants don't require large amounts of land and don't necessitate the extraction or transport of a natural resource from a different region. Nevertheless, there are legitimate environmental concerns regarding the use of geothermal energy for electric power production [Duffield et al., 2005].
Concern over issues such as air pollution, visual and noise effects, surface deformation, local ecology, disposal of hazardous byproducts, and over-tapping hydrothermal systems all must be considered if geothermal energy is to become an environmentally sustainable resource. In most cases, solutions have been developed in order to mitigate these impacts.
Visual Impact
Power plant aesthetics have been taken into account by the Mammoth-Pacific Geothermal plant outside of Mammoth Lakes, California. Designers have been praised for blending this power plant so well into its natural surroundings that motorists traveling down the adjacent highway are typically unaware of the plant's existence! [http://www.mammothpacific.com/awardfacilities.html]
Harmful Gases
The release of greenhouses gases such as dissolved carbon dioxide (CO 2 ) does occur in geothermal power production (excluding binary systems), though the amount released is considerably less than the gases produced by coal plants of similar sizes [Mock et al., 1997]. To some, the use of isobutane in binary systems may be more concerning. According to Jim Shallcross (engineer at the Mammoth-Pacific Geothermal plant), there is enough isobutane in the M-P binary plant to fill an Olympic-sized pool. If this amount of isobutane were to explode, it would affect an area within a two mile radius [J. Shallcross, pers. comm., 2006].
Hazardous Waste Byproducts
Another important environmental hazard associated with the Mammoth-Pacific plant, and many other geothermal plants, is the production of hazardous waste sludge containing heavy metals, salts and toxic minerals. These byproducts precipitate out of brine solutions within the piping networks of geothermal plants and, when removed, they are considered hazardous waste and can't be disposed of in normal waste streams. though efforts are being developed to remove toxic elements from the waste. Returning this toxic waste from whence it came (the ground) could be risking, depending on the existence of clean-water aquifers. Efforts are being made, however, to remove hazardous materials from this waste [Goethermal Today, 1999].
Surface Deformation
Subsidence of surface areas over a tapped hydrothermal reservoir can occur when geothermal fluids are reinjected at different rates and in different areas than where they originally came from. The effects of landform deformation have been studied by the USGS in the Casa Diablo well field (where the Mammoth-Pacific plant is located). Results show that “subsidence may result in part from reductions in fracture spacing and slippage along faults,” due in part to the disruption of natural hydrothermal circulation, but also to the change of temperature within the hydrothermal reservoir [Sore et al., 1995].
Depletion of Reservoir
Geothermal energy's status as a sustainable resource is generally uncontested, but considering it a renewable resource is another story. In order for geothermal energy to be considered renewable, special care must be taken to ensure that the resource is properly managed to maintain pressure and productivity over a long period of time. Mock et al. [1997] explains that sustaining a hydrothermal system requires that its rate of consumption does not exceed its rate of generation; in other words, caution must be expressed so as not to over-tap any given geothermal reservoir.
The Future of Geothermal Energy in the U.S. and Abroad
Mock et al. [1997] argues that social and economic progress is dependent on sufficient energy supplies at acceptable costs. On the global scale, many developing countries could benefit from their geothermal resources by producing sustainable energy.
Indonesia, for example, sits on a hot bed of geothermal reserves, which could be used to produce more than 20,000 megawatts worth of electricity. Instead, leaders in the country are relying on their existing supply of fossil fuel resources, which are currently more economically viable [ http://www.csmonitor.com/2004/1209/p07s02-woap.html].
In the United States, exploitable geothermal energy resources are only available on the west coast, and thus can only provide a small percentage of the country's energy needs. If geothermal energy is to become available everywhere as a major energy source, it is suggested that hot dry rock systems be utilized [Mock et al., 1997]. Hot dry rock (HDR) systems are high-temperature rock fields located at drillable depths everywhere that could be used for direct-use applications or even for the generation of electricity [Duffield and Sass, 2005].
The potential for obtaining energy from HDR systems is enormous, but only if technology allows the implementation of man-made hydrothermal circulation systems in hot dry rocks [Wohletz and Heiken, 1992]. Until proper heat mining technology is available and the need for geothermal development is great enough, this vast resource cannot be successfully developed on a commercial scale.
Conclusion
The value of geothermal energy is undeniable – geothermal development has proven that the Earth's energy can be harnessed to produce clean, sustainable power in a number of ways. Developing nations can experience economic and social progress by utilizing their own geothermal resources and, with the arrival of new technologies, this energy source will play an even bigger role in the United States. So, as fossil fuels continue to be depleted and global warming becomes a threat that can't be ignored, this sustainable resource will undoubtedly gain more prominence in the years to come.
Sources
Decker, R., and B. Decker, 1998, Volcanoes . New York: W.H. Freeman Press.
Duffield, W., and J.H. Sass, 2003, Geothermal Energy – Clean Power From the Earth's Heat . U.S. Geological Survey Circular, No. 1249.
Hulen, J.B. and P.M. Wright, 2001, Geothermal Energy: Clean Sustainable Energy for the Benefit of Humanity and the Environment . Energy and Geoscience Institute, University of Utah .
Keller, E.A., 2000, Environmental Geology. New Jersey: Prentice Hall.
Mock, J.E., J.W. Tester, and P.M. Wright, 1997. Geothermal Energy From the Earth: Its Potential Impact as an Environmentally Sustainable Resource . Annual Reviews Energy Environments, 1997.
Sore, M.L., C.D. Farrar, G.A., Marshall, and J.F. Howell, 1995, Effects of Geothermal Development on Deformation in the Long Valley Caldera, 1985-1994 . Journal of Geophysical Research.
Wohletz, K., and G. Heiken, 1992, Volcanology and Geothermal Energy. Berkeley and Los Angeles: University of California Press.
Wright, P.M., The Sustainability of Production From Geothermal Resources, in Geo-Heat Bulletin, Vol. 19, No. 2 article.
Geothermal Today: Clean Energy for the 21 st Century, Geothermal Energy Program Highlights, U.S. Department of Energy report, 1999. * No author credited. *