During the last several millennia, the area now comprising the Long Valley Caldera to Mono Lake has been volcanically active. During past activities of the various volcanoes in the Long Valley-Mono Lake area there have been many volcanic hazards. Some of the activities that create these hazards are still occurring even today, though, not nearly as many hazards are present as once were in the region.
Volcanic activity in the Mono Lake-Long Valley region began about 15- 8 Ma in what is currently the Aurora-Bodie area. This volcanic field is east-northeast of Mono Lake. This first period of activity included calc-alkaline andesite, dacite and trachyandesite lavas, breccias, and ashflow tuffs. Above this layer is a slightly younger layer comprised alkaline-calcic cinder cones and their associated flows. This layer is packed much more tightly than the older, underlying layer [Wood and Kienle, 1990].
The next concentration of activities occurred between 4.5 to .25 Ma. The era from about 4.5-2 Ma saw andesite domes and flows, concentrated near the Cedar Hill region, as well as cinder cones and flows comprised of late Holocene basalts. Also at this time and in this area was the Mud Springs volcano. This now appears as a steep, bulbous flow encompassing a sunken vent. The Aurora Crater only came into being approximately .25 Ma. This crater is surrounded by lava flows and also shows evidence of some of the same basalts as near Cedar Hill [Wood and Kienle, 1990].
Left: Picture 1.1 Aerial view of the Mud Springs Volcano, (south up). Picture from Wood and Kienle, 1990.
Right: Picture 1.2 Aerial view of the Aurora Crater, (southeast up). Picture from Wood and Kienle, 1990.
Volcanic activity in the present day Long Valley region did not manifest itself until approximately 3.5 Ma. The largest part of those first eruptions was the extensive basaltic lava flows. Magma with a slightly higher silica level was also present, though in much lower quantities; these were andesite as well as some quartz latites. Much of this first phase of activity in the region can still be seen in the eroded remnants of the Adobe Hills and the High Sierras [Harris, 1988].
Over the next millennia a magma chamber began to grow beneath what is now the Long Valley Caldera. This magma was extremely high in silica and therefore prone to highly explosive eruptions. This silica rich magma first erupted between 2.1 to 8 Ma producing massive rhyolitic deposits. This formed the present day Glass mountain region [Harris, 1988].
It was approximately 700 Ka that the most influencial eruption occurred. This was the eruption that created the Long Valley Caldera and greatly shaped the surrounding area. The magma from this eruption was rhyolitic, high in both silica and gas, which accounts for the explosive eruption that took place. Due to the high silica content, the magma was highly viscous, which means that the gas would have had a hard time escaping from it. This magma rose in fractures that were in the crust. As the magma rose and broke towards the surface, the pressure that was holding the gas in solution was finally released. When that happened, the gas expanded rapidly resulting in a massive explosion that ejected ash, lava, and tephra [Harris, 1988].
Pyroclastic flows poured down the sides of the volcano, westward, and reached to at least the present day San Joaquin River , if not further. Another wave of pyroclastic flows seems to have headed more south towards the present day Owens Valley . These pyroclastic deposits, on top of the airfall deposits of ash and pumice, layered to create what is now called the Bishop Tuff. Central California to southwest Nevada was also heavily layered with airfall deposits of ash and lapilli, as well as some pyroclastic deposits with pumice, though these did not reach quite as far. Due to the explosivity of the Long Valley eruption, a recognizable layer of ash reached as far east as Nebraska . When the eruption finally ended, the magma chamber was so emptied that the roof of the volcano collapsed into the area that once housed the magma. This formed the present day Long Valley Caldera [Harris, 1988].
Simply because that eruption ended with the collapse of the volcano, this did not mean that all volcanic activity in the area suddenly ceased. On the contrary, the area resumed activity almost immediately, geologically speaking. Between 500 to 600 Ka, the caldera floor began to show signs of volcanism. Further explosions, though smaller than the major eruption that had just taken place, ejected tephra from the floor high into the air. This was followed by flows of obsidian forming rhyolite. During this time, magma began to rise towards the surface again, forming the Resurgent Dome approximately 600 Ka. Eruptions of highly silictic rhyolitic magma continued on and off at about 200,000 year intervals. These collected in the ‘moat' around the Resurgent Dome inside the Caldera, starting about 500 Ka [Harris, 1988].
Mammoth Mountain , on the southwest edge of Long Valley , added to the region's activity with its own eruption approximately 150 Ka. This mountain erupted almost every 5,000 years over a period of about 100,000 years. Evidence of this can be seen today in the 20 or so overlapping domes and lava flows that now make up the mountain. Around this time, activity connected to the Mono-Inyo chain also began. It started approximately 200 Ka with basaltic lava flows that covered the area from the Devil's Postpile to Black Point [Harris, 1988].
Map of Mono Lake- Long Valley Region.Picture from Hill et al., 1985, found in Wood and Kienle, 1990.
Activity actually at the present day Mono-Inyo craters began some time after that, about 35 Ka, marking what some consider to be the beginning of the second phase of, “the development of large mid-crustal magma chambers and associated calderas [Wood and Kienle, 1990]” in the region. The arch of the Mono Craters reaches from Mono Lake to the edge of the Long Valley Caldera. The Mono chain consists largely of three main obsidian flows, six to eight rhyolitic domes, and many craters. While the oldest date to approximately 35 Ka, most of them are as recent as the last 10,000 years [Wood and Kienle, 1990]. Approximately 30 vents have appeared in the last 2,000 years alone. Black Point is also included in the Mono chain. The maze of basaltic fissures, on the north side of Mono Lake , was created about 13.5 Ka as part of an underwater eruption [Harris, 1988]. A basaltic tephra cone, also on the north shore, resulted as well. The islands in Mono Lake , Negit and Paoha, make up the last bit of the Mono chain. They also represent that third and newest phase of, “the development of large mid-crustal magma chambers and associated calderas [Wood and Kienle, 1990].” These islands are only about 1,800 years old. The bigger island of Paoha even has ash and lava dated to only about 200 years old [Wood and Kienle, 1990].
Map of Inyo Craters, showing domes, flows, and phreatic craters. Picture from Map of Sampson, 1987, in GSA Spec. Pap. 212, 89-101, found in Wood and Kienle, 1990.
The Inyo chain, which stretches from the Mono chain, south into the Long Valley Caldera, is relatively young, even compared to the Mono chain. This chain's activity only started about 6 Ka. The majority of the chain's activity is only about 600 years old. This is from around the same time that Panum Crater and its associated flow-banded rhyolitic dome formed in the Mono chain. Much of the Inyo activity, due to wet fill in the caldera, produced only phreatic craters. However, some magma rose outside the caldera, in drier climes, and created domes and lava flows. The tip of the Inyo chain incorporates some phreatic craters on the south slope of Mammoth Mountain. These last expulsions on Mammoth Mountain were indeed phreatic and occurred approximately 500 years ago [Wood and Kienle, 1990].
After Mount St. Helens erupted in 1980, there were swarms of quakes in the Mono Lake- Long Valley region. Near the Casa Diablo Hot Spring, new steam vents also opened; this was in 1984. Also not long after Mt. St. Helens , the resurgent dome showed signs of growth [Harris, 1988]. Today, near the Horseshoe Lakes around Mammoth Mountain , there are massive tree kill areas. Often times in a volcanically active region, there can be high carbon-dioxide levels. Here the levels are so high in the soil that they are suffocating the tree roots. This kills the trees and leaves visible evidence of possible volcanic activity in the region. So, even today, the activity continues.
Given the high amount of activity during this era, there were, of course, many volcanic hazards. The severity of some of these hazards was in part due to the type of magma being erupted. One of the most obvious hazards to most people is, of course, lava. Lava is molten rock, magma, which has finally broken through to the surface. The hazards from lava can vary based on its composition. Basaltic lavas are composed of a lower quantity of silica, 45-55%, and tend to erupt very effusively. The lava has a low viscosity, so it flows fairly easily. It also has a relatively low gas content. These things combine to make for a rather quiet eruption. Basalt erupting volcanoes are less explosive because the low viscosity molten rock does not trap as much gas, what little gas there is in this lava. So, there is less tephra being ejected into the air from these. Basaltic lava can flow farther than the other two types, making the lava itself more dangerous to forests, wildlife, houses, and other man-made structures. However, they do not pose a grave threat to humans, as most people could out run a basaltic lava flow [Hill, 1975]. These sorts of flows make up several early pieces of the Mono Lake-Long Valley region. The Cedar Hill region has evidence of some basaltic flows [Wood and Kienle, 1990]. Some of the earliest Long Valley eruptions were also extensively basaltic, as were the Black Point Fissure eruptions [Harris, 1988].
Andesitic lava is considered an intermediary type. Its silica content is right in the middle, about 55-65%, making it slightly more viscous than basalt. The gas content is also higher, making these eruptions more prone to explosivity than effusion. As a result, there is a wide variety of tephra, from ash-bombs. Ejecta can also be thrown higher and farther making the threat from ejecta greater. The lava can pose some danger to the area directly around the volcano, but as the viscosity is higher, the lava can not flow as far as basaltic lava can. Quite a bit of the current Sierra Nevada area is made up of andesitic flows [Hill, 1975]. The Aurora- Bodie area has andesite flows [Wood and Kienle, 1990]. Long Valley , in its middle eruptive stage, was also highly andesitic [Harris, 1988].
Rhyolitic lava is the last type. The silica content in this is extremely high, 65-75%. This makes for an intensely viscous lava that often traps the higher gas content. This usually leads to violent explosive eruptions. Many cubic feet of ejecta are produced and flung far and wide. Ash from such an eruption can circle the globe and can affect everything from sunsets to climates. The high viscosity though, means that there is not nearly as much danger directly from the lava. The lava can not make it very far, and therefore usually affects only the immediate volcanic area [Hill, 1975]. The explosions from the gas trapped in the lava, though, are very violent. It is these eruptions that often produce calderas like Long Valley . Most of the Mono-Inyo chain is also made up of rhyolitic flows, domes, and tephra rings [Harris, 1988].
While all three of these lava types produce slightly different hazard levels from lava, they also spew forth, at least some, ejecta or tephra. The smallest form of tephra is considered to be dust. This is followed by ash, lapilli, and bombs. These titles each represent a different size of tephra. The size also determines how far the ejecta can travel. The table below shows the relationship.
Size |
Name |
Distance |
< 1/8 mm |
volcanic dust |
1000's km-global |
1/8- 2mm |
volcanic ash |
10's- 100's km |
2mm-6cm |
lapilli |
1- 10 km |
>6 cm |
bombs |
< 1km |
Table 1.1- [Sizes from Decker and Decker, 1998. Distances from the Lecture notes of Michael Hamburger, 2004. Names from both.]
Basaltic volcanoes are not very explosive, but even they can produce tephra. Some slight ash and little lapilli are usually as big as it gets [Prager et al., 2000]. Andesitic volcanoes are more explosive and can produce anything from ash – bombs. Though, these eruptions are not usually violent enough to launch the ash world wide [Decker and Decker, 1998]. Rhyolitic volcanoes, though, possess the power needed to launch the ash and dust globally. They also can produce the biggest, farthest traveling bombs. Ash can also cause damage. It can be aspirated and cause bodily harm, and when mixed with water ash becomes extremely heavy and often collapses roofs. Ash on the ground means that plants die. Lapilli and bombs, of course, can cause impact damage to trees, wildlife, or any human structures that might be in the area [Decker and Decker, 1998].
The varying power behind the eruptions, that these lavas influence, also affects two other hazards, lahars and pyroclastic flows. For some reason, eruptions with andesitic lava are more prone to lahars, volcanic mud flows. Mud and debris mixed with volcanically hot water can move extremely fast. However, if the debris and mud is mixed with cooler water, from glaciers or mountain streams, the flow can move more slowly [Hill, 1975]. Another way to characterize lahars are as cohesive and noncohesive debris flows. Cohesive flows have more clay in them and are extremely think for their entire run. Noncohesive flows have less clay and depend on high velocities to keep them thick [Decker and Decker, 1998]. Either way, these mud flows tend to hug the lowland areas, stream beds, and valleys. They can cover a great distance and when they harden some have been as thick as sixty feet [Hill, 1975].
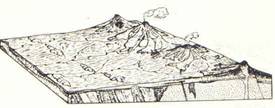
What Hill postulates the Sierra Nevadas may have looked like after the inundation of lahars. Picture from Hill, 1975.
The high explosivity of rhyolitic eruptions, means that they are more prone to giant pyroclastic flows[Hill, 1975]. Pyroclastic flows are often broken down into several types: Pumice flows, nuées ardents, and pyroclastic surges. Pumice flows leave behind unconsolidated deposits of pumice. The pumice usually varies in size and the deposits are usually called ignimbrites. Nuées ardents leave behind various fragments that are denser than pumice. These can range in size from ash- small bombs. Pyroclastic surges are the flows of hot ash and gas. They carry with them fragments that are less dense than those carried by pumice flows or nuées ardents. Together, these three things make up the official pyroclastic flow. These flows are essentially a mass of hot ash and gas, and small debris too dense to make it all the way off the ground. Instead of becoming fully airborne, these ‘clouds' hug the ground and lowlands. They can travel at least 100 kilometers per hour and can sterilize upwards of 400 square kilometers around an eruption area. These flows leave pyroclastic deposits in their wake [Decker and Decker, 1998].
Despite the fact that the different lava compositions can lead to slightly different volcanic hazards, there are some hazards that don't readily depend on the type of lava to determine their existence. Harmonic tremors are one such thing. These tremors are low frequency swarms, which usually indicate magma movements upward towards the surface. Some larger earthquakes can also occur in an attempt to relieve some of the pressure in the highly charged volcanic area. Both of these types of quakes can cause damage to man-made structures, affect wildlife, or even open fissures in the ground which might then trigger a full volcanic eruption as gas is able to come out of solution [Decker and Decker, 1998]. The fissures that magma was able to rise in prior to the apocalyptic eruption of Long Valley may have been caused by such quakes. Currently, there are still small quakes in the Long Valley Caldera, which could show possible activity [Harris, 1988].
Phreatic Explosions are another hazard. When magma rises into an area where there is either a high water table or an area with wet fill, this changes what happens. Instead of erupting, the magma will be quenched by the water. This results in a phreatic, or steam, explosion followed by some ash. Several of the Mammoth Mountain craters are a result of this [Wood and Kienle, 1990].
Gas emissions can be another volcanic hazard. Volcanically active areas often have high emissions of carbon-dioxide coming out of volcanic vents. CO 2 is colorless, odorless, heavier than air, and can suffocate without warning [Decker and Decker, 1998]. When CO 2 emitted from the magma becomes trapped underground, it will often find its way to the surface through fractures or faults. It hugs the low lying ground because it is heavier than air. It can collect in valleys, basements, and other low areas and kill without warning. This is currently the case near Mammoth Mountain where the high CO 2 is slowly killing the trees in the area [Sorey et al., 2000].
The Mono Lake- Long Valley Region has seen much volcanic activity in the past millennia. With this activity it has also seen the hazards that go with it. Some of these activities, such as quakes and gas emissions, are still occurring in the area. They are still posing hazards for the area as well. Other volcanic hazards, though, such has lahars, pyroclastic flows, and roaring rivers of molten rock, seem to be behind Mono Lake and Long Valley …for now.
Works Cited
Decker, Robert and Barbara Decker. Volcanoes: Third Edition . New York : W.H. Freeman and Company, 1998.
Hamburger, Michael. “Volcanic Hazards.” 2004 Earthquakes and Volcanoes-G141. Indiana University , Bloomington . Fall of 2004.
Harris, Stephen. Fire Mountains of the West: the Cascade and Mono Lake Volcanoes . Missoula : Mountain Press Publishing Company, 1988.
Hill , Mary . Geology of the Sierra Nevada . Berkeley : University of California Press, 1975.
Prager, Ellen et al. Furious Earth. New York : McGraw-Hill, 2000.
Sorey, Michael et al. U.S.G.S.: Invisible CO 2 Gas Killing Trees at Mammoth Mountain , California . Revised Reprint by the U.S.G.S w/ Pennsylvania University , 2000.